ACL injuries receive a tremendous amount of attention at all levels of sport, and for good reason. It is estimated that 250,000 ACL injuries occur annually in the United States, representing approximately $2 billion in medical and rehabilitation costs.1 Many of these injuries occur in competitive athletes, with females demonstrating a particularly high risk for ACL injury in field- or court-based sports (e.g., soccer, lacrosse, basketball). The occurrence of a single ACL injury may result in many future complications, including reduced activity levels, cessation of sport participation, and a high risk for future ACL injuries.2
So, what do we currently know about ACL injury?
Without rehashing too much of a previous post on ACL mitigation strategies, most of the research and clinical practice has focused on biomechanical- and neuromuscular-based interventions. While these programs have been demonstrated to be successful3, the rates of ACL injuries are actually climbing in adolescents4 and remaining somewhat steady in professional athletes (figure 1).5
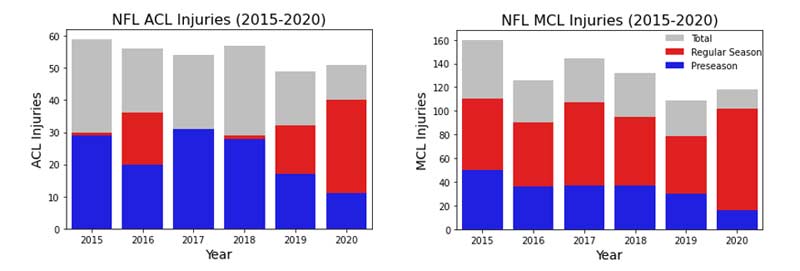
In this blog post, I share what we currently know about the sensorimotor contributions to ACL injury risk, as well as how we can utilize technology- and field-based training strategies to improve sensorimotor performance in our athletes.
*Author’s note: I define sensorimotor performance as the integration between perceptual sensory input (e.g., vision, hearing, touch) and biomechanical movement output (e.g., running, jumping, cutting, decelerating). There are varying definitions, but for the sake of clarity, this is my working definition for the remainder of the post.
A New View of ACL Injuries?
As you may know, many individuals—myself included—have devoted entire careers to understanding ACL injuries and strategies to mitigate this injury risk. My doctoral research examined the relationship between sports-related concussion and lower extremity/ACL injury risk in adolescent and collegiate athletes. With concussive injuries being classically defined by a temporarily altered sensorimotor state, I started to wonder how these attributes contributed to ACL injury (outside of athletes with a recent or past concussion history).
Previous research has identified biomechanical and neuromuscular contributors to ACL injury risk (dynamic knee valgus, quadriceps-to-hamstring strength ratio).6 However, this is not necessarily conclusive in all athlete populations.7,8 While it is outside the scope of this article to discuss the extreme complexity of ACL injury, my current research has led me to believe that perhaps we are somewhat ignoring another important contributor to ACL injury…the brain!
My current research has led me to believe that perhaps we are somewhat ignoring another important contributor to ACL injury—the brain, says @JasonAvedesian. Share on XUltimately, the central nervous system (we can generally think of this as the brain and spinal cord) is the central driver of biomechanical and neuromuscular control. Before getting into the actual research, I want to take you along a theoretical approach to how the brain and sensorimotor performance may contribute to ACL injury.
Adding the Brain and Sensorimotor Performance to the ACL Injury Risk Equation
Let’s first give some thought to the actual complexity of a dynamic sporting environment. Take, for example, a collegiate midfield soccer athlete performing in a very intense match. Some of the key sensorimotor attributes to successful performance and staying injury-free may include:
- Working memory and pattern recognition – Remembering and recognizing the opposition’s defensive scheme when in a certain position on the field.
- Dual-tasking – Receiving the ball from their teammate while scanning the field.
- Visual attention and multiple object tracking – Spatial recognition of the changing position of teammates and opponents.
- Reaction time and processing speed – Avoiding oncoming defenders.
All of this must be completed within hundreds of milliseconds! When viewed from this perspective, we truly take for granted the complexity of sporting environments (and how athletes can make their performances in them look rather “effortless”). Any movement performed on the field requires a complex interaction between the environment and athlete (we can think of this as sensorimotor integration).
Basically, any interaction an athlete makes with their environment is based upon three general concepts: the nature of the information, the complexity of the action to be performed, and the number of available response options (figure 2). Regardless of the task, movement behavior is a constantly evolving process constrained by time, space, and decisions (figure 2).
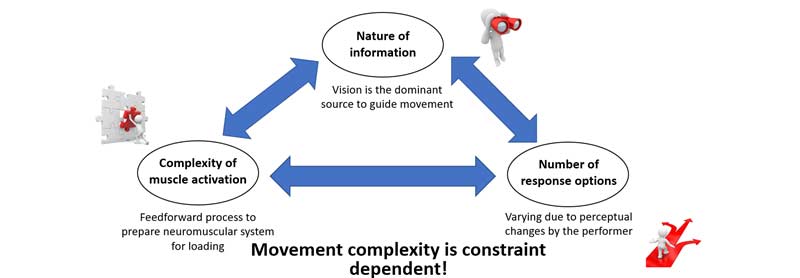
What Does the Current Research Tell Us?
Much like examining movement biomechanics and muscular activity patterns, what if we could determine how an athlete’s central nervous system is performing? Luckily, researchers have initiated these studies in the hopes of quantifying sensorimotor performance as it relates to ACL injury risk. In this section, I will highlight some studies that have been conducted, along with areas for future research and sports science directions.
One of the first studies on the relationship between sensorimotor performance and ACL injury risk was conducted by Swanik and colleagues all the way back in 2007.9 In this study, the researchers collected preseason performance on a common concussion assessment (ImPACT) and then longitudinally tracked non-contact ACL injuries in a group of collegiate athletes. Compared to athletes of the same sport and position, athletes who sustained an in-season, non-contact ACL injury performed worse on all measures of the assessments, including reaction time, processing speed, and working memory.9
Follow-up studies in collegiate football athletes have demonstrated an association between worse visuo-motor reaction time and greater risk for non-contact lower extremity injury.10,11 A recent investigation from one of my colleagues (Dr. April McPherson of the USOPC) determined that individuals post-concussion are at a 1.6x greater odds for an ACL injury compared to those without a concussive injury history.12 Hot off the press last month, researchers determined that female lacrosse athletes are at a fivefold increased risk for ACL injury up to one year post-concussion.13
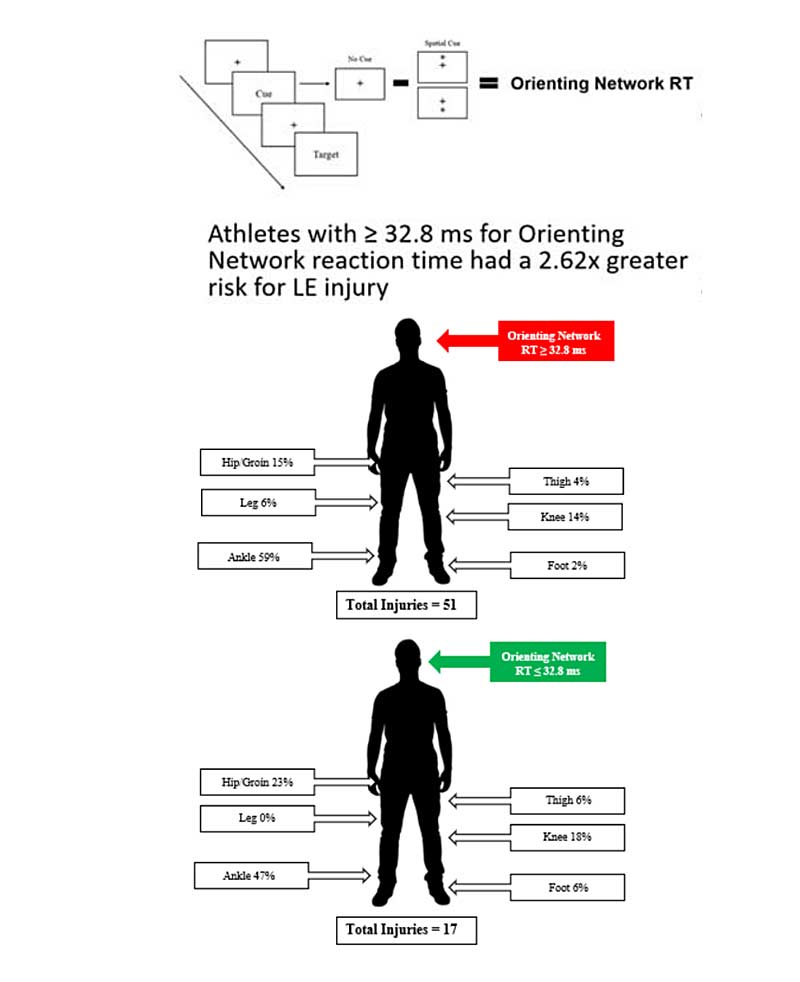
Compared to more established biomechanical and neuromuscular data, quantifying the influence of sensorimotor performance on ACL injury risk is relatively lacking and still very much in its infancy. From what we do know so far, however, there are three brain areas that may be most influential to sensorimotor performance and, in turn, risk for ACL injury.
- Prefrontal cortex – Responsible for many roles, including information processing/filtering, executive function, and working memory performance.
- Thalamus – Acts as a relay of motor and sensory signals to the cerebral cortex for higher-level processing.
- Lingual gyrus – Responsible for visual perception and recognition of complex vision information.
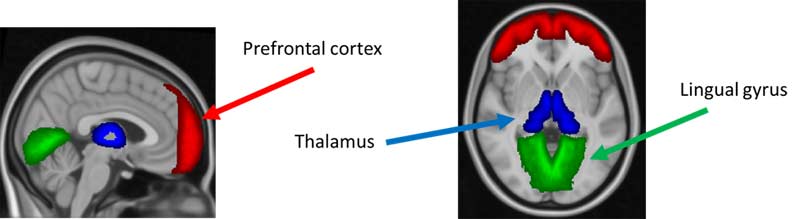
My overall conclusion of the sensorimotor research to date is that athletes with relative deficits in sensorimotor performance may be more susceptible to ACL and lower extremity injuries during conditions of increased arousal (i.e., a sporting environment). While certainly more research is required (and there’s a lot of exciting work coming down the pipeline), we can at least begin to ask: how can we train the sensorimotor system?
Athletes with relative deficits in sensorimotor performance may be more susceptible to ACL and lower extremity injuries during conditions of increased arousal (i.e., a sporting environment. Share on XTraining the Sensorimotor System – Technological Advancements
Over the last decade, there have been some major advancements in “train the brain” technology. Devices and software such as sensory boards, reactionary light devices, stroboscopic eyewear, and virtual/augmented reality* attempt to target various sensorimotor performance attributes: visual spatial-attention and reaction time, working memory, pattern recognition, and multiple object tracking (figure 5).
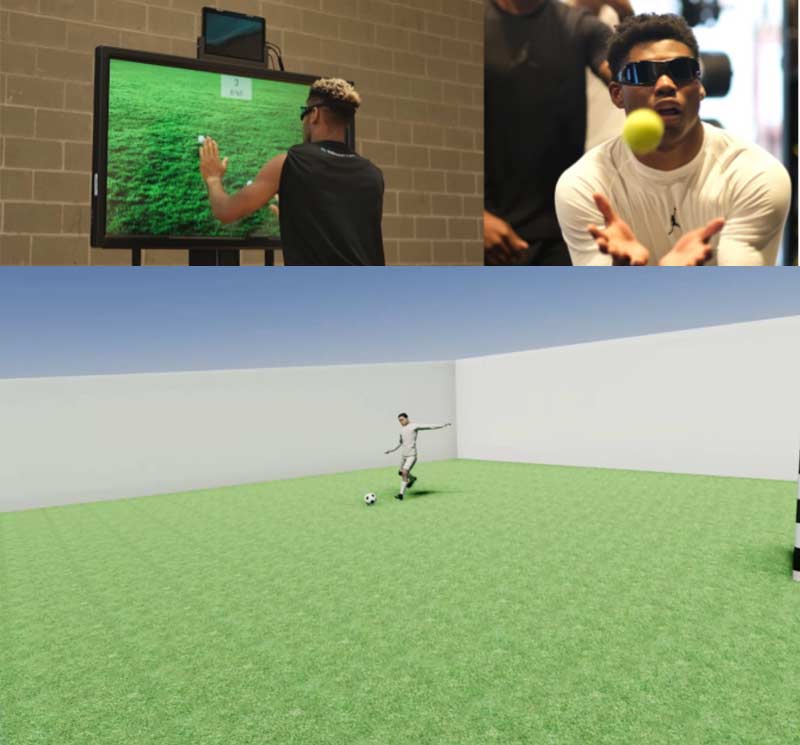
*Disclaimer: This blog post is not intended to be an endorsement of any one product. I am just sharing my personal experiences with these devices, along with some research-grade evidence to support their potential utility.
Before we decide to invest in new technology, let’s consider a recent paper from Hadlow and colleagues14 on the framework for utilizing sensorimotor technology (figure 6).
Based on the Hadlow model, there are three considerations when implementing new technology to train the sensorimotor system.
- Targeted Perceptual Function – The ability to discriminate between athletes of various skill levels (e.g., professional athletes should perform better than adolescent athletes).
- Stimulus Correspondence – Sensorimotor skill improvement should improve through targeted training (e.g., visual-spatial attention and processing speed should improve with stroboscopic eyewear training).
- Response Correspondence – Trained sensorimotor skills should translate to enhanced on-field performance and reduced injury risk.
Of these three factors, response correspondence is the least studied in the sports science literature. However, it offers plenty of opportunities for researchers and sports scientists to explore how current sensorimotor technology/training strategies influence performance and injury risk. In the next sections, I will take a deeper dive into a few of these sensorimotor technologies.
Sensory Board Technologies
Sensory board training devices have become a popular tool for assessing and training a variety of sensorimotor abilities. From a sports science standpoint, here are a few considerations to collect the most accurate data from these devices:
- Standardize the time of assessment – Sensorimotor performance can be affected by many internal and external stressors, including sleep quality15, anxiety levels16, fatigue17, and prior injury18. Therefore, longitudinal sensorimotor measures should be collected at the same time of day to minimize these effects. For example, it would not be ideal to collect a baseline preseason assessment at 6 a.m. and compare that to future assessments collected in the mid-afternoon during the season.
- Standardize board height and distance – Most existing sensory board technology is based upon upper extremity sensorimotor performance. During each assessment, athletes should be placed at standardized locations relative to their height and arm length to ensure accurate comparisons across time. This standardization will be helpful in minimizing effects on central and peripheral visual reaction time.
- Standardize attentional focus – This is the toughest standardization to implement without actual eye tracking technology. Devices such as Dynavision and Senaptec Sensory Station offer central fixation targets during certain sensorimotor assessments. It is important to instruct athletes to fixate on these targets, especially if the sports scientist or researcher is interested in collecting peripheral visual-spatial performance.
While sensory boards are a bit more costly than other sensorimotor technology, these devices can assess and train many important attributes such as eye-hand and eye-foot coordination, central and peripheral visual-spatial attention, multiple object tracking, working memory, and reaction time. Research indicates that worse performance on sensory boards is associated with greater risk for lower extremity injury in collegiate football athletes.19 Even if we do not have access to sensory board technologies, there are other options for assessing and training sensorimotor abilities.
Stroboscopic Eyewear
Stroboscopic technology within elite sport has been around for quite some time (check out this article dating back to MJ in his prime). Nowadays, the technology is very easy to use and implement within a sport and clinical setting (figure 7). The goal is to limit visual information by alternating between clear and opaque visual states while performing sport-specific activities. This technology may be particularly helpful for athletes who are over-reliant on vision (often the case in athletes during rehabilitation from lower extremity injury such as ACL20) by re-weighting sensory input to vestibular and somatosensory systems during dynamic postural control tasks.20
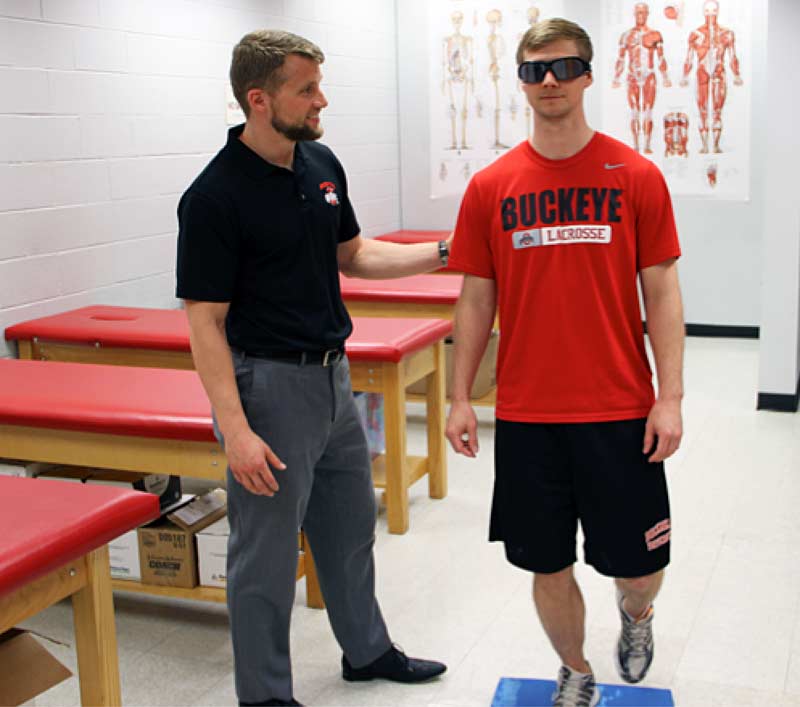
There are several sensorimotor abilities that may be trained when using stroboscopic eyewear, including external attentional focus, visual-motor processing speed for enhanced visual efficiency, working memory, anticipatory trajectory estimation (e.g., ball flight, oncoming opponent), and transient visual attention.21,22 While this technology certainly requires more research and data, it appears that beneficial training effects may be seen in as little as three weeks.23
Virtual Reality
Sports-specific virtual reality (VR) is another up-and-coming technology that will begin to permeate through training environments. In one of my previous stops at the Emory Sports Performance and Research Center (in partnership with Cincinnati Children’s Hospital), the research team developed sports-specific scenarios integrated within biomechanical analysis to better understand ACL injury risk during more realistic conditions.
The utilization of VR for sensorimotor training comes with many potential benefits for practitioners and clinicians:
- VR offers fully immersive environments within clinical and/or laboratory settings for better replication of sports-specific demands.
- Practitioners gain the ability to simultaneous collect neuromuscular and biomechanical outcomes related to ACL injury risk during VR assessments.
- Similar to stroboscopic eyewear, utilizing VR may help an athlete transition from a predominately internal attentional focus to an external attentional focus during training.
Training the Sensorimotor System – Revisiting Technological Advancements
Overall, there are many emerging technologies that can be used to train the sensorimotor system. While the research and data still relatively lag clinical practice (spoiler: they usually do), there is certainly utility for all the previously discussed technologies. When revisiting the Hadlow framework14, it appears that stroboscopic eyewear and sports-specific VR may be the most effective devices for training, while sensory board technology may be best for standardized assessments and monitoring change over time (figure 8).
Stroboscopic eyewear & sports-specific VR may be the most effective devices for training, while sensory board technology may be best for standardized assessments and monitoring change over time. Share on XTo reiterate, much more sports science research is required on all these technologies, but I am confident that we will see much more of that over the next few years. My advice to clinicians and sports scientists looking to invest in sensorimotor technology: first determine how feasible it will be to implement within your athletes’ specific training environment. Once you decide that, you will be able to make the appropriate choice(s) that fit your needs.
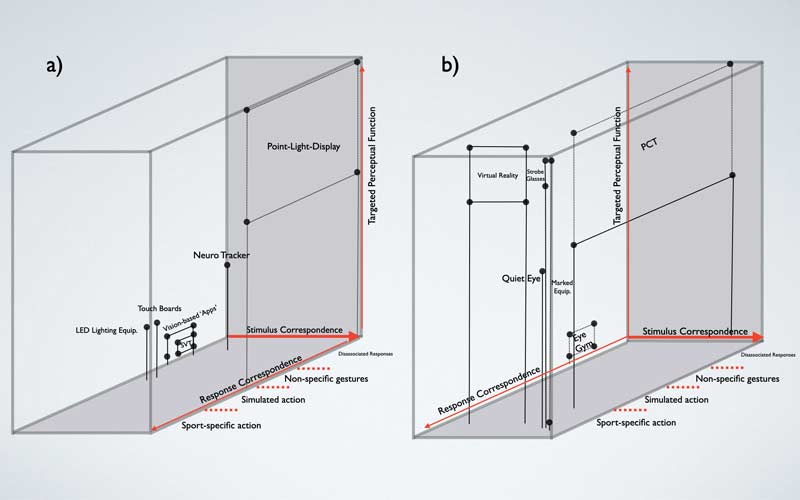
While we all love technology and the great strides it has made for training our athletes, we must all consider more “field-based” training strategies. In this next section, I will discuss how we can capitalize on agility training to develop sensorimotor abilities.
Agility vs. Change of Direction
Before diving too deep, I must first briefly discuss the differences between agility and change of direction (COD) training. While both have merit within a training model, it is important to distinguish between the two to target desired outcomes. As defined by Sheppard and Young (2006)24, agility is a rapid, whole-body movement in response to a stimulus, while COD is a rapid, whole-body movement that is pre-planned. Keep in mind, COD qualities are instrumental for agility performance. But when examining the two from a sensorimotor perspective, there are important differences:
- Agility attributes – Anticipation, visual-spatial attention, pattern recognition, visuo-motor processing speed, and reaction time.
- COD attributes – Technique, linear/horizontal speed, neuromuscular asymmetry, and eccentric and deceleration control.
The environment we place our athletes in may also be quite different when targeting agility versus COD attributes. Agility-based training is more random and chaotic (open-skill abilities), while COD training tends to be more controlled and pre-planned (closed-skill abilities). COD training is inherently stable, while agility situations present an unstable environment in which an athlete is under various demands/constraints from teammates and opponents, all while having to anticipate and make decisions under time and space constraints (see figure 2).
While all athletes can benefit from both styles of training, novice athletes or those in the early stages of injury rehabilitation should be initially placed in COD environments and then progressed to agility environments. The figure I created below provides a quick overview of the important aspects of agility and COD (figure 9).
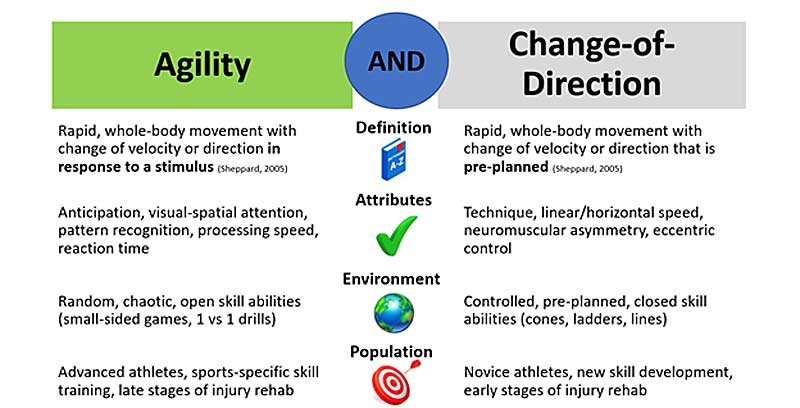
Much like neuromuscular strength training, we can progress agility training to place greater sensorimotor demands on our athletes. Check out a previous blog post in which my colleague Corey Peterson (University of Minnesota) provides a great progression from COD training to agility training. There are near infinite possibilities with agility training.
Determine the sensorimotor demands placed on your athletes and mimic your training environment to better replicate those demands, says @JasonAvedesian. Share on XThe bottom line: determine the sensorimotor demands placed on your athletes and mimic your training environment to better replicate those demands.
Sensorimotor Considerations During Injury Rehab
Unfortunately, we will not be able to prevent all lower extremity/ACL injuries from occurring in sports. In the event of an ACL injury, we must be aware of the sensorimotor contributions during rehabilitation so that we can reduce the risk of future injury. As we know, ACL injury rehab can be very complex and may not follow precise timelines due to setbacks. In the sub-acute, acute, and even chronic stages of post-ACL injury, physiological responses such as pain, stiffness, and swelling may occur.25,26 This may lead to ruminating-type behaviors and psychological distress appearing in the form of anxiety, fear of reinjury, and decreased confidence to return to previous performance levels.27
We can think of this psychological response as simply stress that athletes must manage while returning from injury. High stress levels are associated with delayed reaction times28, reduced attention capacity29, and internal attentional focus30, all of which can be thought of as sensorimotor deficits (figure 10).
Aside from restoring neuromuscular and biomechanical performance capacities, we must consider the restoration of psychological and sensorimotor abilities during ACL injury rehab. The previously mentioned technologies are fantastic tools for these exact purposes, especially early in the rehab progression when athletes may not be able to get full “physical reps.”
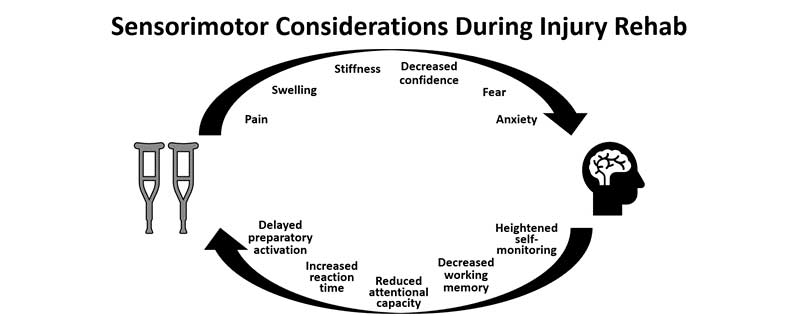
*Author’s note: If you are interested in the actual brain neurophysiological response to an ACL injury, I would highly recommend checking out the work of my colleague Dr. Dustin Grooms at Ohio University. He has done tremendous work in this space and has been greatly influential on my current understanding of the sensorimotor contributions to ACL injury.
Relationship Between Concussion and ACL Injury
Much of my personal motivation on sensorimotor contributions to ACL injury came from my PhD studies at UNLV and Michigan State University. My dissertation research focused on how sports-related concussion and neurocognition influenced lower extremity biomechanics and injury risk in adolescent and collegiate athletes.31–34 Prior data has determined that athletes and military personnel are at approximately 2–3 times greater risk for lower extremity injury post-concussion (figure 11).35–39
As mentioned earlier, recent research indicates a specific relationship between concussion and ACL injury risk.12,13 Transient deficits in cognition and oculomotor performance are hallmark signs of a concussive injury, but researchers are beginning to think that more subtle sensorimotor deficits may still linger even after athletes have been cleared to return to sport. The previously discussed sensorimotor topics in this blog post can certainly apply (and perhaps be very effective) during the acute and chronic time periods post-concussion to mitigate future risk for lower extremity and ACL injuries.
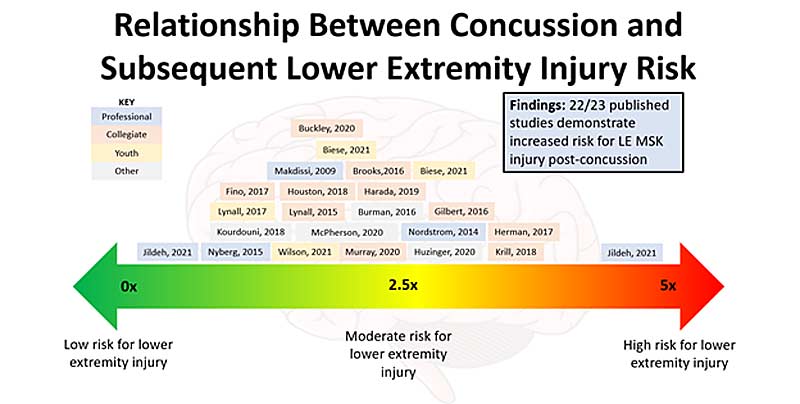
Concluding Thoughts – Future Opportunities to Reduce the Risk of ACL Injury
While we know quite a bit about ACL injuries from biomechanical and neuromuscular perspectives, we still have much to discover in terms of how sensorimotor performance contributes to ACL injury risk. With the research and information I have gathered at this stage in my career, here is my proposed pathway to ACL injury (figure 12):
- Cascading events that begin with decreased visual-spatial attention, delayed reaction time/processing speed, and/or reduced working memory.
- Perception-action mismatch between the athlete and surrounding environment (e.g., mistimed estimation of a defender’s trajectory toward the athlete).
- Delayed neuromuscular response (e.g., anticipatory quadriceps and hamstring response) that results in increased load on the knee joint during high-impact loading events.
- Increased risk for ACL injury.
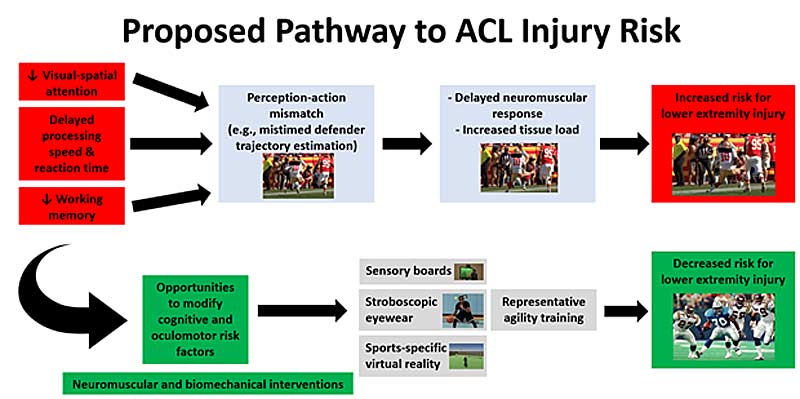
If you take one thing away from this entire blog post, I hope it is that we have a great opportunity to modify sensorimotor risk factors and incorporate sensorimotor training within previously established neuromuscular and biomechanical interventions to reduce the risk of ACL injury.
Header photo by Tony Quinn/Icon Sportswire.
Since you’re here…
…we have a small favor to ask. More people are reading SimpliFaster than ever, and each week we bring you compelling content from coaches, sport scientists, and physiotherapists who are devoted to building better athletes. Please take a moment to share the articles on social media, engage the authors with questions and comments below, and link to articles when appropriate if you have a blog or participate on forums of related topics. — SF
References
1. Bates NA, McPherson AL, Rao M, Myer GD, and Hewett TE. “Characteristics of inpatient anterior cruciate ligament reconstructions and concomitant injuries.” Knee Surgery, Sports Traumatology, Arthroscopy. 2016;24(9):2778-2786.
2. Paterno MV, Rauh MJ, Schmitt LC, Ford KR, and Hewett TE. “Incidence of contralateral and ipsilateral anterior cruciate ligament (ACL) injury after primary ACL reconstruction and return to sport.” Clinical Journal of Sport Medicine. 2012;22(2):116-121. doi:10.1097/JSM.0b013e318246ef9e
3. Webster KE and Hewett TE. “Meta-analysis of meta-analyses of anterior cruciate ligament injury reduction training programs.” Journal of Orthopaedic Research. 2018;36(10):2696-2708. doi:10.1002/jor.24043
4. Beck NA, Lawrence JTR, Nordin JD, DeFor TA, and Tompkins M. “ACL tears in school-aged children and adolescents over 20 years.” Pediatrics. 2017;139(3). doi:10.1542/peds.2016-1877.
5. Injury Data Since 2015. NFL.com. Accessed August 7, 2021. https://www.nfl.com/playerhealthandsafety/health-and-wellness/injury-data/injury-data
6. Hewett TE, Myer GD, and Ford KR. “Anterior cruciate ligament injuries in female athletes: Part 1, mechanisms and risk factors.” American Journal of Sports Medicine. 2006;34(2):299-311. doi:10.1177/0363546505284183
7. Cronström A, Creaby MW, and Ageberg E. “Do knee abduction kinematics and kinetics predict future anterior cruciate ligament injury risk? A systematic review and meta-analysis of prospective studies.” BMC Musculoskeletal Disorders. 2020;21(1):563. doi:10.1186/s12891-020-03552-3
8. Krosshaug T, Steffen K, Kristianslund E, et al. “The Vertical Drop Jump Is a Poor Screening Test for ACL Injuries in Female Elite Soccer and Handball Players: A Prospective Cohort Study of 710 Athletes.” American Journal of Sports Medicine. 2016;44(4):874-883. doi:10.1177/0363546515625048
9. Swanik, Covassin T, Stearne DJ, and Schatz P. “The relationship between neurocognitive function and noncontact anterior cruciate ligament injuries.” American Journal of Sports Medicine. 2007;35(6):943-948.
10. Wilkerson GB. “Neurocognitive reaction time predicts lower extremity sprains and strains.” International Journal of Athletic Therapy and Training. 2012;17(6):4-9.
12. Wilkerson GB, Simpson KA, and Clark RA. “Assessment and training of visuomotor reaction time for football injury prevention.” Journal of Sport Rehabilitation. 2017;26(1):26-34.
13. McPherson AL, Shirley MB, Schilaty ND, Larson DR, and Hewett TE. “Effect of a Concussion on Anterior Cruciate Ligament Injury Risk in a General Population.” Sports Medicine. 2020; 50(6):1203-1210.
14. Lutz RH, DeMoss DJ, Roebuck EH, Mason T, and Eiler BA. “Sport-Specific Increased Risk of Anterior Cruciate Ligament Injury Following a Concussion in Collegiate Female Lacrosse.” Current Sports Medicine Reports. 2021;20(10):520-524. doi:10.1249/JSR.0000000000000839
15. Hadlow SM, Panchuk D, Mann DL, Portus MR, and Abernethy B. “Modified perceptual training in sport: A new classification framework.” Journal of Science and Medicine in Sport. 2018;21(9):950-958. doi:10.1016/j.jsams.2018.01.011
16. LaGoy AD, Ferrarelli F, Sinnott AM, Eagle SR, Johnson CD, and Connaboy C. “You Snooze, You Win? An Ecological Dynamics Framework Approach to Understanding the Relationships Between Sleep and Sensorimotor Performance in Sport.” Sleep Medicine Clinics. 2020;15(1):31-39. doi:10.1016/j.jsmc.2019.11.001
17. Nieuwenhuys A and Oudejans RRD. “Anxiety and perceptual-motor performance: toward an integrated model of concepts, mechanisms, and processes.” Psychological Research. 2012;76(6):747-759. doi:10.1007/s00426-011-0384-x
18. Pageaux B and Lepers R. “The effects of mental fatigue on sport-related performance.” Progress in Brain Research. 2018;240:291-315. doi:10.1016/bs.pbr.2018.10.004
19. Busch A, Blasimann A, Mayer F, and Baur H. “Alterations in sensorimotor function after ACL reconstruction during active joint position sense testing. A systematic review.” PLoS One. 2021;16(6):e0253503. doi:10.1371/journal.pone.0253503
20. Grooms D, Appelbaum G, and Onate J. “Neuroplasticity following anterior cruciate ligament injury: a framework for visual-motor training approaches in rehabilitation.” Journal of Orthopaedic & Sports Physical Therapy. 2015;45(5):381-393. doi:10.2519/jospt.2015.5549
21. Kim KM, Kim JS, Oh J, and Grooms DR. “Stroboscopic Vision as a Dynamic Sensory Reweighting Alternative to the Sensory Organization Test.” Journal of Sport Rehabilitation. 2020;30(1):166-172. doi:10.1123/jsr.2019-0466
22. Appelbaum L and Erickson G. “Sports vision training: A review of the state-of-the-art in digital training techniques.” International Review of Sport and Exercise Psychology. 2016;11:1-30. doi:10.1080/1750984X.2016.1266376
23. Appelbum LG, Cain MS, Schroeder JE, Darling EF, and Mitroff SR. “Stroboscopic visual training improves information encoding in short-term memory.” Attention, Perception, and Psychophysics. 2012;74(8):1681-1691. doi:10.3758/s13414-012-0344-6
24. Shekar SU, Erickson GB, Horn F, Hayes JR, and Cooper S. “Efficacy of a Digital Sports Vision Training Program for Improving Visual Abilities in Collegiate Baseball and Softball Athletes.” Optometry and Vision Science. 2021;98(7):815-826. doi:10.1097/OPX.0000000000001740
25. Sheppard JM and Young WB. “Agility literature review: classifications, training and testing.” Journal of Sports Science. 2006;24(9):919-932. doi:10.1080/02640410500457109
26. Filbay SR and Grindem H. “Evidence-based recommendations for the management of anterior cruciate ligament (ACL) rupture.” Best Practice & Research: Clinical Rheumatology. 2019;33(1):33-47. doi:10.1016/j.berh.2019.01.018
27. Wang B, Zhong JL, Xu XH, Shang J, Lin N, and Lu HD. “Incidence and risk factors in joint stiffness after Anterior Cruciate Ligament reconstruction.” Journal of Orthopaedic Surgery and Research. 2020;15(1):175. doi:10.1186/s13018-020-01694-7
28. Meierbachtol A, Obermeier M, Yungtum W, at al. “Injury-Related Fears During the Return-to-Sport Phase of ACL Reconstruction Rehabilitation.” Orthopaedic Journal of Sports Medicine. 2020;8(3):2325967120909385. doi:10.1177/2325967120909385
29. Tomczyk CP, Shaver G, and Hunt TN. “Does Anxiety Affect Neuropsychological Assessment in College Athletes?” Journal of Sport Rehabilitation. 2020;29(2):238-242. doi:10.1123/jsr.2018-0123
30. Oudejans RRD, Kuijpers W, Kooijman CC, and Bakker FC. “Thoughts and attention of athletes under pressure: skill-focus or performance worries?” Anxiety Stress Coping. 2011;24(1):59-73. doi:10.1080/10615806.2010.481331
31. Mullen R, Faull A, Jones ES, and Kingston K. “Attentional Focus and Performance Anxiety: Effects on Simulated Race-Driving Performance and Heart Rate Variability.” Frontiers in Psychology. 2012;3:426. doi:10.3389/fpsyg.2012.00426
32. Avedesian JM, Covassin T, Baez S, Nash J, Nagelhout E, and Dufek JS. “Relationship Between Cognitive Performance and Lower Extremity Biomechanics: Implications for Sports-Related Concussion.” Orthopaedic Journal of Sports Medicine. 2021;9(8): 23259671211032250. doi:10.1177/23259671211032246
33. Avedesian JM, Covassin T, and Dufek JS. “Landing biomechanics in adolescent athletes with and without a history of sports-related concussion.” Journal of Applied Biomechanics. 2020;(Jul 31):1-6. doi:PMID:32736349
34. Avedesian JM, Covassin T, and Dufek JS. “The influence of sport-related concussion on lower extremity injury risk: A review of current return-to-play practices and clinical implications.” International Journal of Exercise Science. 2020;13(3):873-889.
35. Avedesian JM, Forbes W, Covassin T, Dufek JS. “Influence of Cognitive Performance on Musculoskeletal Injury Risk: A Systematic Review.” American Journal of Sports Medicine. Published online March 19, 2021: 363546521998081. doi:10.1177/0363546521998081
36. Brooks MA, Peterson K, Biese K, Sanfilippo J, Heiderscheit BC, and Bell DR. “Concussion Increases Odds of Sustaining a Lower Extremity Musculoskeletal Injury After Return to Play Among Collegiate Athletes,” American Journal of Sports Medicine. 2016;44(3):742-747.
37. Fino PC, Becker LN, Fino NF, Griesemer B, Goforth M, and Brolinson PG. “Effects of Recent Concussion and Injury History on Instantaneous Relative Risk of Lower Extremity Injury in Division I College Athletes.” Clinical Journal of Sports Medicine. 2019;29(3):218-223.
38. Harada GK, Rugg CM, Arshi A, Vail J, and Hame SL. “Multiple Concussions Increase Odds and Rate of Lower Extremity Injury in National Collegiate Athletic Association Athletes After Return to Play.” American Journal of Sports Medicine. 2019;47(13):3256-3262.
39. Lynall RC, Mauntel TC, Pohlig RT, and al. “Lower Extremity Musculoskeletal Injury Risk After Concussion Recovery in High School Athletes.” Journal of Athletic Training. 2017;52(11):1028-1034. doi:10.4085/1062-6050-52.11.22
40. McPherson AL, Nagai T, Webster KE, and Hewett TE. “Musculoskeletal injury risk after sport-related concussion: A systematic review and meta-analysis.” American Journal of Sports Medicine.