Muscular power is a skill-related fitness component that many strength and conditioning practitioners look to improve in athletes for success in motor performance. However, as early as the 1980s, the review of sports medicine and human performance literature reveals many different methods of measuring muscular power, not all of which are consistent with its operational definition born from the scholarly discipline of physics (Sapega & Drillings, 1983). While there is an emphasis on developing muscular power to improve motor performance in each athlete’s respective sport, confusion may arise because terminology related to the abstract of power in sport science literature are used interchangeably (Cronin & Sleivert, 2005).
Due to the challenges in interpreting and understanding the performance variable: power, to improve research practice, Cronin and Sleivert (2005) reviewed studies published before 2003 on the influence of maximal power training on improving athletic performance.
Before 2003, it was common in the literature for authors to confuse explosive strength, rate of force development, and impulse as terms synonymous with power (Cronin & Sleivert, 2005). These terms have different and distinct meanings; therefore, the interpretation and applications of the studies’ findings may be misrepresented. This article will define the terminology commonly represented in sport science literature to enable future scientists who choose to research power, rate of force development, or impulse to be clear and consistent when discussing each respective term.
Only when there is a universal understanding of the definitions of power, rate of force development, and impulse is when strength and conditioning practitioners can begin applying the information from the research to develop training programs with clear objectives, such as improving motor performance.
Only when there is a universal understanding of the definitions of power, RFD, and impulse is when S&C practitioners can begin applying the information from the research to develop training programs with clear objectives. Share on XCronin and Sleivert (2005) mention a study (Newton & Kraemer, 1994) that compared different training strategies to increase muscular power but discovered much of their experimental design focused on the development of rate of force development. Another study also looked into the development of power but actually focused on impulse (Hedrick, 1993).
While these two studies aimed to investigate power, they examined rate of force development and impulse, which have different and distinct meanings to power. Therefore, prospective scientists and strength and conditioning practitioners reading their results and findings may take these terms of “power” at face value and overlook the authors’ definitions. For example, a study that measures rate of force development but claims to develop power may be compared to a study that measures impulse but claims to develop power. On paper, the two studies’ interventions aim to increase power, but at its core, they measured two different components that underpin motor performance.
In this case, we cannot and should not compare the two studies as rate of force development and impulse are two variables with different meanings, and making any comparisons would be invalid. Suppose there is a misinterpretation or misrepresentation of findings? In that case, this will affect subsequent studies, and researchers and strength and conditioning practitioners would be in a continuous cycle of being unable to interpret results and draw conclusions from research by other authors.
Therefore, to better draw conclusions from the results and findings from each other (authors and readership), the terminology must be clear and consistent. Additionally, the approach and standardization in the methodology should be considered, which will be discussed later.
With that said, this review aims to:
- Clarify power and related, yet misunderstood terminology.
- Outline all the recommendations and methodological flaws from Cronin and Sleivert’s (2005) review on the challenges in understanding the influence of maximal power training on improving athletic motor performance.
- Construct “general” rules/criterion to determine what is considered a high-quality study in the context of power research and to see if the research done by authors after 2005 have improved their methodology to make their conclusions and claims.
- Apply the above rules in the examination of a commonly occurring discussion amongst strength and conditioning practitioners: whether power cleans are better than trap/hex bar jump squats or vice versa for developing power, thus translating to athletic motor performance.
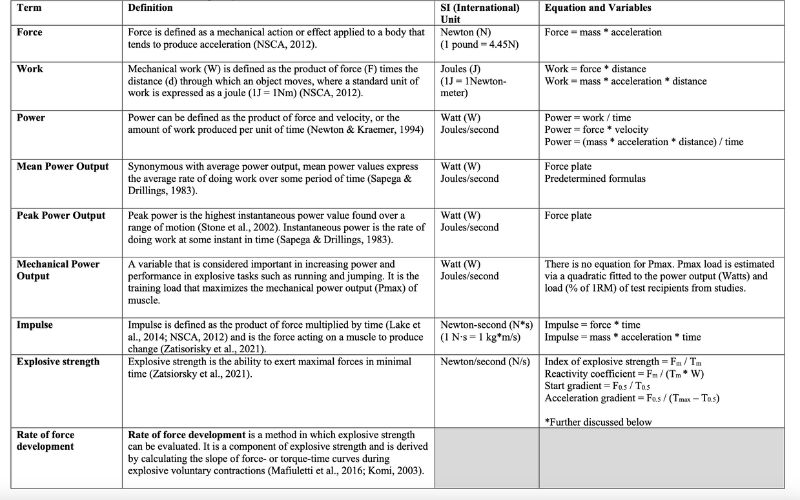
Misunderstood Terminology
Strength and conditioning practitioners looking to develop their athletes should be encouraged to take an evidence-based approach. However, as one searches “power training for athletes” in PubMed or The Journal of Strength and Conditioning Research database, terms such as explosive strength, rate of force development, power, and impulse become apparent in the discussion section of the articles. These terms have different meanings (Table 1), which the authors do not always define.
Therefore, readers who are not experts or lack the foundational knowledge of power may become confused and overwhelmed when attempting to learn about their question of interest. Furthermore, articles that do not distinctly define the terminology may misuse a term. Consistency of terminology amongst researchers and readership regarding scientific dissemination of information is critical so that the approach to the development of athletes is logical and sound. Because there is no consensus with the terminology mentioned above, it has hindered clarity. Search nomenclature in the research database did not always result in what was being looked for and, consequently, has made the investigation into this topic of study difficult.
Consistency of terminology amongst researchers and readership regarding scientific dissemination of information is critical so that the approach to the development of athletes is logical and sound. Share on XAs of 2022, it is difficult to find how the terms explosive strength, rate of force development, and impulse differ from power in one location. One must look through many different resources to fully grasp these terms. To resolve this problem, the goal of this work is to operationalize each term clearly.
Power
Power is defined as the product of force and velocity (distance/time) or the amount of work produced per unit of time (Newton & Kraemer, 1994). Therefore, a powerful individual can produce more work in less time.
For example, consider the following situation:
- Person A: Throws a twenty-pound medicine ball four feet away from the starting point.
- Person B: Throws a twenty-pound medicine ball nine feet away from the starting point.
The mass of the ball between the two individuals is the same. However, Person B is considered more powerful than Person A because Person B can accelerate the mass more and produce greater force into the medicine ball, increasing its velocity and leading to a further distance from the starting point.
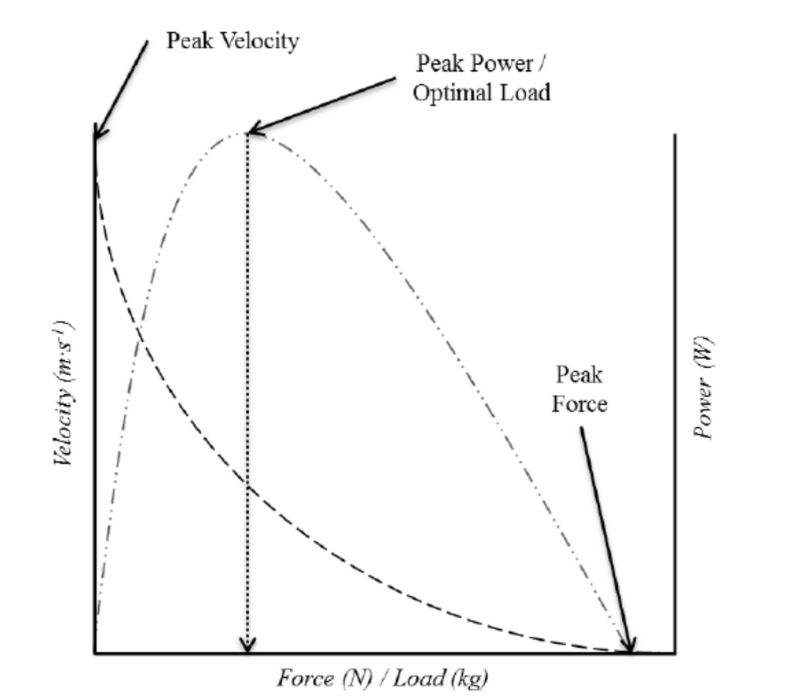
Rate of Force Development
Rate of force development is a component of explosive strength and is derived by calculating the slope of force- or torque-time curves during explosive voluntary contractions (Mafiuletti et al., 2016; Komi, 2003). More specifically, rate of force development is the “force produced from the initiation of the muscle action” (Zatsiorsky et al., 2021). Therefore, an individual with a high rate of force development can produce force faster. For example, when going from a static position to a starting position in a movement, the faster an individual can produce force, the faster their body or limb will move. If we compare two high-level athletes, the individual who can produce a higher rate of force development in a specific movement will likely demonstrate superior motor ability. The “better an athlete’s qualifications, the greater the role the rate of force development can play in terms of [motor] performance” (Zatsiorsky et al., 2021).
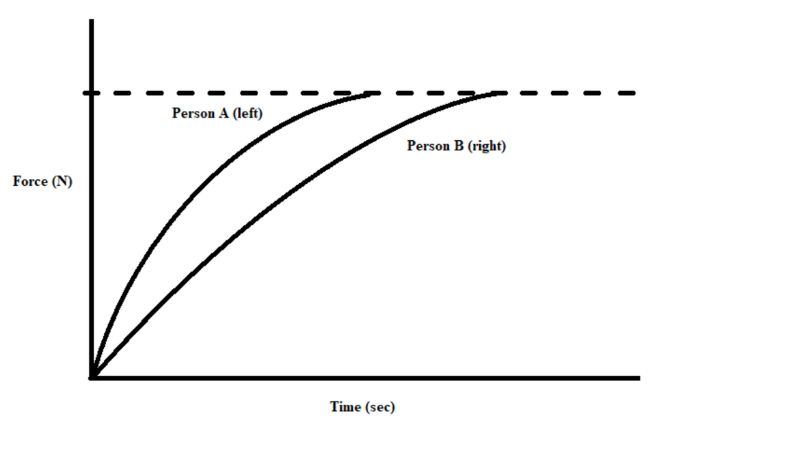
Explosive Strength
Explosive strength is the ability to exert maximal forces in minimal time (Zatsiorsky et al., 2021). It can be considered an “all-encompassing” term. Rate of force development is explosive strength, but explosive strength is not necessarily rate of force development. This is because there are four indices, listed below, used to estimate explosive strength (Zatsiorsky et al., 2021):
- Index of explosive strength (IES)
- IES = Fm / Tm
- Fm is the peak force.
- Tm is the time to peak force.
- This refers to the ability to exert maximal forces in minimal time.
- Reactivity coefficient (RC)
- RC = Fm / (TmW)
- Fm is the peak force.
- Tm is the time to peak force.
- W is an athlete’s weight and expresses the index of explosive strength relative to body mass. Therefore, it is a relative expression (similar to absolute strength vs. relative strength, where absolute strength is the ability to produce maximum force regardless of body weight and relative strength considers body weight).
- RC is typically highly correlated with jumping performances, especially with body velocity after a takeoff.
- Force gradient or Start gradient (S-gradient)
- S-gradient = F0.5 / T0.5
- F5 is one-half of the maximal force Fm.
- T5 is the time taken to achieve one-half of the maximal force Fm.
- The S-gradient characterizes the rate of force development at the beginning phase of a muscular effort.
- Acceleration gradient (A-gradient)
- A-gradient = F0.5 / (Tmax – T0.5)
- F5 is one-half of the maximal force Fm.
- T5 is the time taken to achieve one-half of the maximal force Fm.
- Tmax is the maximum time taken.
- A-gradient is used to quantify the rate of force development in the late stages of explosive muscular efforts.
Impulse
Impulse is defined as the product of force multiplied by time (Lake et al., 2014; NSCA, 2012) and is the force acting on a muscle to produce change (Zatisorisky et al., 2021).
- Impulse = force * time
In summary:
- Power is the work over time OR product of force and velocity.
- Force is the product of mass and acceleration.
- Velocity is the distance over time.
- Explosive strength is the force over time (no distance component).
- Rate of force development is a method in which explosive strength can be evaluated.
- Impulse is the product of force and time.
Examples of Misused Terminology in the Literature
Rate of force development Does Not Equal Power
As Sapega and Drilling (1983) mention, two groups of investigators have used rate of force development as a measure of “power” in their studies which are still cited to this day. By precise definition, calculating rate of force development (force/time) is different from calculating power (work/time). Both studies defined their measure of “power” as force/time, which is in actuality rate of force development.
Therefore, we must be cautious and clearly understand that rate of force development is not equivalent to muscular power output. It becomes an issue if we state that rate of force development is the same variable as power. The rate of force development (force/time) is not a measurement of power, and “any application of the term ‘power’ to muscular performance measurements in (sports medicine literature) that does not represent the correct formulation (force * distance/time, force*velocity or work/time) should be unwarranted” (Sapega & Drillings, 1983).
Peak Power
A group of investigators stated that peak power was calculated by multiplying peak muscular torque by the duration of contraction (Sapega & Drillings, 1983). Again, power is calculated by work/time (i.e., torque * angular displacement /time), not torque/time.
Discussion on Misused Terminology
Now that we have a better understanding and have definitions of how terms are defined, this begs the question: Is power the key performance indicator strength and conditioning practitioners are seeking in the context of motor performance? It has been suggested that for activities that require fast force production (100-300ms), such as jumping and sprinting, rate of force development is the most important determinant of athletic success (Cronin & Sleivert, 2005; Günter & Tidow, 2006).
Moreover, a study by Slawinski et al. (2010) supports this notion as a greater rate of force development was linked to better sprint performance in elite sprinters. Slawinski et al. (2010) suggest that rate of force development contributes significantly to sprinting because “during fast limb movements, the short contraction time may not allow maximal muscle forces to be reached (and therefore), any increase in the contractile rate of force development becomes highly significant because it allows a higher level of muscle force to be reached in the early phase of muscle contraction,” which can act as the distinguishing factor between a faster and slower sprint time.
Furthermore, McLellan et al. (2011) investigated the role of rate of force development on vertical jump performance and suggested that it is strongly correlated to the vertical jump during a counter-movement jump (CMJ). By developing muscular force rapidly (rate of force development), this can increase take-off velocity and in part, be an explanation as to why a significant correlation was observed between rate of force development and vertical jump performance during the CMJ (McLellan et al., 2011). However, it is important to note that the subjects in this study were recreational men with diverse strength levels and training statuses (Table 3). Discussing the underlying physiological attributes of rate of force development is outside the scope of this review. However, in summary, it can be attributed to neuromuscular characteristics such as increased motor unit recruitment, rate coding, motor unit synchronization, and neuromuscular inhibition (Griffin & Cafarelli, 2005; Wiegal et al., 2019).
For activities like squash, badminton, and fencing, the motor performance to quickly complete a lunge and return to the start or move off in another direction is critical for success (Cronin et al., 2003). With this in mind, Cronin et al. (2003) investigated the components that predict lunge performance. Maximal strength, mean power, peak power, and explosive strength were assessed, but explosive strength was the only predictor of lunge performance. The lunge was assessed on the preferred leg via a custom-built supine squat machine where the movement involved a forward lunge (1.5 times leg length) to a marker, and participants returned to the starting position as rapidly as possible (Cronin et al., 2003). Simultaneously, the velocity characteristics of the lunge movement were attained from a linear transducer. Cronin et al. (2003) suggest that producing maximum force earlier in a contraction (greater explosive strength) impacts the velocity characteristics of the lunge motion and increases performance.
Knowing these terms mean fundamentally different things, this begs another question, is one component: explosive strength, rate of force development, peak power, or mean power better than others at predicting a specific athletic motor performance? If so, which components better predict each athletic motor skill (throwing, jumping, sprinting)? Is there a hierarchy or are they equally weighted? We should consider this so that we do not waste resources and the time or energy of athletes because, ultimately, strength and conditioning practitioners want to develop athletes effectively and efficiently.
Knowing these terms mean fundamentally different things, this begs another question: is explosive strength, rate of force development, peak power, or mean power better than others at predicting a specific athletic motor performance? Share on XFor example, Wilson et al. (1995) investigated the relationship between a series of isometric, concentric, and stretch-shortening cycle rate of force development tests performed in an upright squat position to sprint performance. They found that concentric rate of force development was the only component that significantly correlated with sprint performance, and concentric maximum rate of force development was the only measure able to discriminate between good and poor performers. This resulted in authors discussing the “superiority of concentric rate of force development tests over and above isometric and stretch-shortening cycle rate of force development tests and suggest their inclusion in sport science test batteries” (Cronin & Sleivert, 2005).
However, Cronin and Sleivert (2005) highlight that after examining the study, concentric rate of force development and force explained less than 38% variance associated with 30m sprint performance. This indicates that one specific component, in this case, concentric rate of force development, cannot adequately express or provide insight into all mechanisms responsible for 30m sprint performance. Perhaps a combination of power, strength, explosive strength, rate of force development, and impulse will provide the best predictive capabilities of a specific motor performance.
In much of the research, studies tend to either measure rate of force development or power and not both. Perhaps measuring both would be beneficial as we can explain the shared variance associated with these two components associated with motor performance. That way, we can compare and have more concrete evidence to determine which one is better. For example, hypothetically, if rate of force development and power had a shared variance of 38% and 25% to sprint performance, respectively, we could claim that rate of force development is a better predictor of sprint performance. Therefore, we would look into exercise prescription methodology that increases rate of force development which can, in turn, translate to athletic motor performance.
In much of the research, studies tend to either measure RFD or power and not both. Perhaps measuring both would be beneficial as we can explain the shared variance associated with these two components associated with motor performance? Share on XIt may not even be a one size fits all answer. Perhaps there is no tiering system of performance components, and strength and conditioning practitioners need to focus on developing all components of explosive strength (which includes rate of force development), peak power, impulse, and maximal strength to improve athletic motor performance. It may also be that different sport-specific motor skills will have different “component” profiles, and therefore, training will be individualized to improve that aspect. While this has not yet been done, it may be of interest to future research. Conversely, depending on the strength and weaknesses of an athlete, the strength and conditioning practitioner might determine an exercise during an athlete’s training plan that will cater towards the specific motor skill characteristic they must improve. Again, one component does not adequately express or provide insight into all mechanisms responsible for a performance of a task, and it is likely multiple components in conjunction with another explain success in athletic motor performance.
Methodological Flaws Outlined by Cronin and Sleivert (2005)
Cronin and Sleivert (2005) reviewed studies between 1981 and 2003. They looked at loads that maximized mean and peak power output for the upper body, loads that maximized mean and peak power output for the lower body, the relationship between measures of power and performance for the lower body, and studies that have examined the effect of load on power output and performance (Cronin & Sleivert, 2005). Most studies reviewed were from the late 1990s and early 2000s (Table 2). From the review, Cronin and Sleivert (2005) magnify the issues in the fundamental definitions and terminology, common methodological errors, and problems in the choice of motor performance measures by authors, which have led to misinterpretations of research findings.
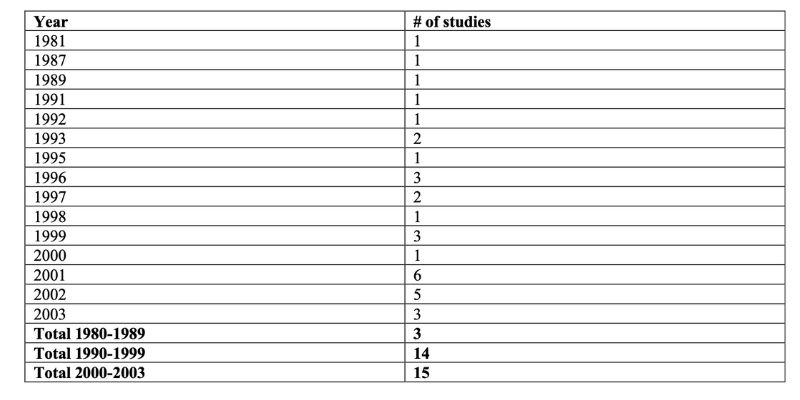
As previously discussed, consistency amongst terminology in all types of research is critical for clear communication between the researcher and readership regarding the scientific dissemination of information. Researchers must be transparent and consistent in their definitions, terminology, and methodological approaches. Furthermore, selecting an appropriate performance measure for the question of interest is critical in designing valid studies. Below are the seven primary methodological flaws outlined by Cronin and Sleivert (2005). Their goal was to eliminate confusion and clarify the type of research needed to advance our knowledge, such that we would “formulate (strong) research designs that result in meaningful and practical information that assists strength and conditioning practitioners in the development of their athletes” (Cronin & Sleivert, 2005).
1. Clear understanding of each research methodology and selecting appropriate performance measures.
With methodological flaws being common in published literature, Cronin and Sleivert (2005) stated a “clear understanding of each research methodology needs to be realized so that the interpretation and application of their finding are not misrepresented.” As such, some studies look at different adaptations or outcomes that are not related to their methods. For this reason, it is pivotal for researchers looking to do their own studies to critically analyze published literature in its totality (not just relying on abstracts) before coming to a conclusion. It is also their responsibility to be clear and transparent in their methodological approach and results. The reader should not have to fill in the blanks and make assumptions because the author chose not to include information.
For example, Schmidtbleicher and Buehrle (1987) compared the effects of three types of training regimes:
- Maximal load (90-100% maximal voluntary contraction (MVC)).
- Power load (45% MVC).
- Hypertrophy load (70% MVC) on various neuromechanical and morphological changes.
An isolated isometric elbow extension movement was used to track these changes. Isometric and dynamic contractions have been shown to differ in physiology and neuromechanics (Wilson & Murphy, 1996). In addition, in terms of athletic motor performance, motor skills are often performed dynamically across several joints in multiple planes. The applicability of an isometric measure is not useful if we are looking to improve athletic motor performance, as it violates the principle of specificity.
2. Research design and combination of training methods.
A common problem in exercise research is having the experimental group receiving multiple variables or treatments simultaneously, making causal interpretations misleading. Many exercise variables can confound one another. In addition, a “robust and rigorous experimental design is one with a control group (which is a group that) receives no treatment, or a standard treatment whose effect is already known” (Leyland & Bott, 2021). Without a control group, the researcher’s ability to draw conclusions about an intervention is greatly weakened. Unfortunately, control groups are typically missing in exercise science research (which may be due to the small sample size), and even if a control group is included, participants not doing any exercise can present a dilemma as “they could lose adaptations, which could make the experimental exercise protocol appear more effective than it really is” (Leyland & Bott, 2021).
When doing exercise research on the athletic population, the sample size in a study can become limited due to extensive inclusion and exclusion criteria which can lead to an underpowered study. As a consequence of an underpowered study, it may have a lower probability of determining the true effect, and in the case of a statistically significant effect, the magnitude may be overestimated and produce false-positive results (Hackshaw, 2008).
Cronin and Sleivert (2005) also highlight that it is common for authors, when studying the effect of load, “to combine training methods which makes the effects of the independent variable impossible to disentangle.” For example, a study investigated the effects of maximal power training (30% 1RM) to heavy load training (75-83% 1RM) combined with plyometric training on a variety of performance measures such as jumping, cycling, and throwing (Lyttle et al., 1996). The maximal power group performed weighted jump squats, and bench press throws, whereas the combined group underwent squats, bench presses, depth jumps, and medicine ball throws. While both training modalities produced significant improvements in performance measures, saying that performance changes were due to the load effect is invalid because we do not know if the improvement was due to the load or the type of exercise performed.
Similarly, Komi et al. (1982) compared the effects of heavy load training to light load training with explosive jump training. Again, due to the experimental group incorporating additional plyometric training, observed performance changes in this study cannot be attributed to the load effect.
3. Training status of subjects.
Training status or age in the context of resistance training, defined as an individual’s training experience and background, can be classified into three components (Table 3): novice, intermediate and advanced (Leyland & Bott, 2021).
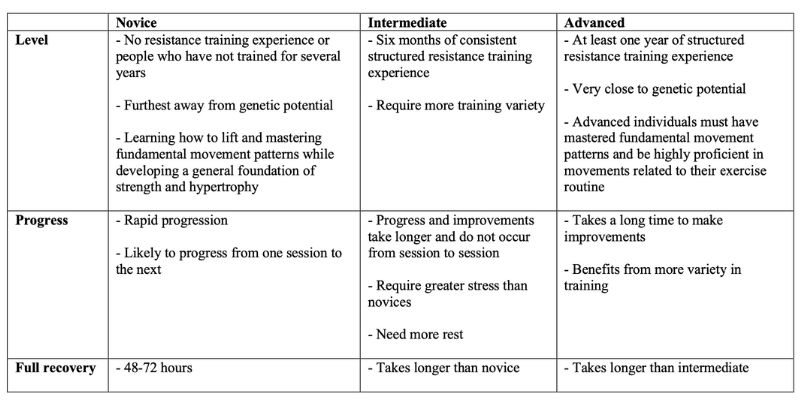
Some studies used novice weightlifters or students as subjects, as they are more easily accessible. This becomes an issue because the research findings may be compromised due to the trainability of a novice subject, thereby affecting the validity of the results. For example, strength can increase rapidly in untrained individuals due to neural adaptations (Griffin & Cafarelli, 2005). Voluntary activation of motor neurons is enhanced due to the activation of previously inactive motor units and the increased rate coding frequency (Griffin & Cafarelli, 2005). An untrained individual relative to a trained individual will have a greater pool of inactive motor units, and therefore, even if a training modality led to significant improvement in an untrained individual (novice), it does not mean it will translate to a highly trained individual (advanced). It could be that the stress applied from training to the untrained individual was sufficient to elicit adaptations, but it does not meet the required threshold to elicit adaptations in a trained individual. For this reason, with respect to athletic motor performance, a study’s sample size should be representative of the population of interest and not utilize untrained subjects, as it limits the generalizability to athletic populations.
With that said, when research studies in sports science rightfully use athletes as their subjects, it is common to see subjects identified as elite, professional, or semi-professional. However, there may be ambiguity in how these terms are defined across authors. To make comparisons across studies, it will be beneficial to see how authors define and classify their subjects with respect to the nomenclature or title they assign.
4. Failure to equate loading.
In exercise, volume load is an estimate of the amount of work accomplished and considers both the training load and the training intensity. It can be expressed as the total product of load (kg), sets performed, and the number of repetitions for each set (Leyland & Bott, 2021). External loading can be quantified quite simply; thus, reporting on load should be a straightforward process (Leyland & Bott, 2021). As Cronin and Sleivert (2005) mentioned, “equating by volume is the most common method by which research compares the effect of load on various (performance) measures.”
- Volume Load = Load * Sets * Reps
In research, many studies have failed to equate loading across training protocols. As a result, it is “difficult to interpret as the reported differences between various training protocols may be contaminated by differences in training volume, rather than the kinematic and kinetic characteristics of different loading intensities” (Cronin & Sleivert, 2005).
For example, an often-quoted study (Wilson et al., 1993) compared traditional weight training (6-10RM squats), plyometric training (unloaded depth jumps), and explosive weight training at the load that maximized mechanical power output (jump squats) to examine their effects on strength and power outputs. Wilson et al. (1993) concluded that after ten weeks, explosive weight training yielded the best overall results because there were significantly greater results in the countermovement jump (17.6%) and static jump (15.2%) compared to traditional weight training (5.1% and 6.8%), and plyometric training group (10.3% and 7.2%).
Despite the detailed and strict testing procedures outlined by Wilson et al. (1993), they fail to specify the volume load of each training group. Perhaps the explosive weight training group performed with greater loads, reps or sets than the traditional weight training and plyometric training groups, which is the reason why Wilson et al. (1993) observed significantly greater results. Ultimately, since the authors failed to equate loading, it is impossible to disentangle the training effects, and therefore the reader must make assumptions. For this reason, researchers must be more detailed and forthcoming in their methodology concerning training volume load when making conclusions about the efficacy or adaptations of various training protocols on performance measures. Studies that do not equate loading in some manner should be cautiously approached, as their findings are likely contaminated.
5. Movement pattern vs. loading intensity.
Another aspect to consider when changes in performance are seen between groups is the effects of the movement pattern. For example, on their first day learning a complex movement like the clean and jerk, an individual will lack coordination. However, through training practice, new motor patterns are learned, and the individual will become more efficient in the movement over time.
Concerning Wilson et al.’s (1993) study, perhaps the explosive weight training group (jump squats) did not produce the best countermovement and static jump results due to optimal load, but rather jump squat training offers greater movement pattern specificity than more traditional strength-training methods. This, perhaps, allowed the subject to unintentionally practice and become familiarized with the movement for the countermovement and static jump test. Jump squat training is described as a method of ballistic strength training and is “thought to be superior to traditional strength-training methods as the velocity and acceleration/deceleration profiles better approximate the explosive movements used in athletic performance” (Cronin & Sleivert, 2005). For this reason, researchers should clearly understand the movement patterns and kinematics of the movement in question, factor in motor skill learning and consider the effects on the results they obtain.
6. Test batteries: type of isoinertial assessment used in training studies.
When comparing training groups, assessments must “balance between being specific to the functional task whilst being sufficiently different from training so that it does not advantage (one group over the other)” (Cronin & Sleivert, 2005). Furthermore, if strength and conditioning practitioners want to improve athletic performance, the testing battery should be specific and applicable to their sport and movement of interest.
For example, if one training group is doing the squat while another is doing the leg press and the 1RM squat is assessed, it can be expected that the results will heavily bias the squat group. If the assessments are incongruent with the loads or exercises the subject is exposed to, it will be difficult to make any sort of conclusion or determination due to the principle of specificity (Leyland & Bott, 2021). The principle of specificity states that a specific type of exercise will elicit specific adaptations that lead to specific training effects (Leyland & Bott, 2021). Therefore, assessments should relate to the training the subject is exposed to. It is also ideal to standardize assessments across a range of loads to remove bias across groups.
7. Establishing Pmax.
Maximum power output (Pmax) is the training load that maximizes the mechanical power output of the muscle. Based on the studies that Cronin and Sleivert (2005) reviewed, Pmax was selected based on previous research, meaning the same Pmax was assigned to the entire group. No research paper prior to 2003 had established Pmax for their respective subjects. This is an issue because there are discrepancies in research regarding which load maximizes power output during various resistance exercises. Therefore, training and testing all subjects at one universal load (ex. 30% 1RM) is fundamentally flawed due to interindividual Pmax differences, which are attributed to factors such as training status (strength level) and the exercise (muscle groups used). In fact, this is fundamentally flawed in all sport science research as it violates the principle of individuality. Due to interindividual differences, each individual Pmax needs to be determined, monitored, and adjusted such that the subjects train at this load to see the true effects of what is being studied.
For example, a commonly cited study (Kaneko et al., 1983) chose to examine the effects of 3 isotonic loads (0%, 30%, 60% of 1RM) on Pmax and found that the load at 30% was most effective in improving Pmax. However, the design does not mean that 30% of 1RM is the load that maximizes power output. Rather, the maximized power output could be anywhere between 30-60% 1RM. Nevertheless, many authors misinterpret these findings and cite this study as support for light isotonic loads (30%) producing maximal mechanical power output.
In addition, when studying power-load relationships, we must be cautious as some research examines the power-load relationship indirectly by investigating the relationship without reporting the load that maximized power output (Cronin & Sleivert, 2005). For example, Cronin and Sleivert (2005) mention a study (Mastropaolo, 1992) where power outputs across a load range of 20-100%1RM were measured in the bench press and without statistical analysis, Mastropaolo (1992) concluded that the load maximizing power output occurred at 40% of 1RM. Not only did Mastropaolo (1992) make a claim without statistical analysis, but Cronin and Sleivert (2005) also observed that the data for power output was very similar across 40-60% of 1RM. It could be that the load maximizing power output ranges anywhere between 40-60% of 1RM, but we cannot make clear justifications without statistical analysis.
Scientists should avoid making assumptions and ensure the results’ interpretations are not misrepresented. By preventing the spread of misinformation, it will ensure the integrity of subsequent future research.
Other Cautions to Consider in the Literature
In addition to the seven methodological flaws, Cronin and Sleivart (2005) eloquently outlined considerations that must be followed when drawing conclusions from the literature on this topic of study. The cautions are discussed below.
8. Different modes of dynamometry.
Another issue that makes the comparison between studies complex is the different modes of dynamometry (isometric, isokinetic, and isoinertial) used to measure strength and power. Isokinetic and isometric assessments have “little resemblance to the accelerative (and) decelerative motion implicit in limb movement during resistance training and athletic motor performance” (Cronin & Sleivert, 2005). Moreover, those who train to increase power will have limited or no access to isometric and or isokinetic dynamometry, and therefore, when comparing research, isoinertial (constant gravitational load) research should be the focus (Cronin & Sleivert, 2005). This speaks to the sixth point mentioned above in relation to the principle of specificity. Performance-related fitness improvements will be specific to the type of exercise training performed, and therefore, training should be “done in a manner as close as possible to how you wish to use its benefits” (Leyland & Bott, 2021).
9. Uniarticular motion vs. multiarticular motion.
Some studies attempt to examine power through uniarticular, or single joint motion. However, athletic motor skills requiring high power outputs such as sprinting, jumping, and throwing are performed by multiple joints. If we are looking to improve these motor skills, research should focus on investigating the power-load spectrum using dynamic (isoinertial) multiarticular motion analysis.
10. Mixed terminology.
As seen in the terminology section (Table 1), the term “power” has been applied too broadly in the sport science literature, creating an identity problem for the term. Therefore, scientists and strength and conditioning practitioners who look to utilize this term, whether in research or an outcome measure in athletes, must be clear and consistent in its true definition.
11. Variety of mathematical methods are used to calculate power.
Due to the variety of methods used to calculate power output, inter-individual comparisons between studies become impossible. For example, in some studies, body mass is used to calculate loading intensity for ballistic motions such as jump squats because the subject must propel themselves and the bar. However, other studies have excluded body mass from the equation, decreasing the total mass component of force and decreasing total power output. It is then up to the reader to extrapolate this data (if even possible) before making comparisons. For literature to be easily digestible and applicable, a standard method for calculating power in resistance training movement needs to be agreed upon.
12. Diversity in testing procedures.
All the following examples are from Cronin and Sleivert’s (2005) review:
- Studies measure power via different modalities of work
- Modalities of work can differ greatly. Some examples may include the following:
- Margaria Kalamen step test (cyclic stretch-shortening cycle test to calculate anaerobic power).
- Treadmill sprint test (cyclic stretch-shortening cycle assessment).
- Continuous jumping protocol (cyclic stretch-shortening cycle assessment).
- Wingate test (cyclic in nature but does not involve stretch-shortening cycle motion).
- Diversity is seen in testing procedures even when the same test is used:
- For example:
- In Wingate tests, studies calculated power output over different time periods with different loads.
- Variety in motor performance measures
- There does not seem to be an all-encompassing motor performance measure that all studies look at. For example, motor performance measures in Cronin and Sleivert’s (2005) review include 5m sprint time, 10m sprint time, 40m sprint time, 100m sprint time, 40-yard dash, and vertical jump.
All in all, research that has investigated power development and related components, such as rate of force development up until 2003, is represented by a great deal of variation in methodology. These variations make comparisons difficult, and therefore definitive conclusions are impossible. This leads strength and conditioning practitioners in directions that may not be best practice.
Inclusion Criteria of Studies
Below is a Power Research Checklist (Table 4) that highlights the methodological flaws mentioned by Cronin and Sleivert (2005). For this paper, the checklist was used to determine the quality of research done after 2005 to see if the methodology actually followed Cronin and Sleivert’s (2005) recommendations. Using this checklist will help the reader assess the quality of the research and will help determine if the authors’ conclusions and claims are appropriate for the implementation of specific methods and exercises in an athlete’s training program. The checklist itself was influenced by the PEDro scale, which was developed to determine the quality of clinical trial literature in physiotherapy evidence (de Morton, 2009).
Because of the vast number of methodological flaws, as Cronin and Sleivert (2005) have mentioned, it is without question that one may be skeptical about the validity of the studies conducted before 2003. However, it is also gravely important to see if studies after 2005 have followed Cronin and Sleivert’s (2005) recommendations. By applying and understanding Cronin and Sleivert’s (2005) recommendations, research designs will be robust and result in meaningful and practical information that strength and conditioning practitioners can utilize in developing their athletes. If research designs have continued to violate Cronin and Sleivert’s (2005) recommendations, it will call attention to how science in this topic of study has not moved forward since 2005 and that awareness must be made for the integrity of future research.
For the final installment of this series and the analysis of ‘power and its elusive identity,’ we chose to utilize the checklist (Table 4) and investigate this question: Are power cleans superior to trap/hex bar jump squats (HBJS) or are trap/hex bar jump squats superior to power cleans as a modality to improve power? This has been a commonly debated topic amongst strength and conditioning practitioners. Albeit with very little substantiation. Yet, we will argue, that many have not gone down this path or really trying to determine what precisely we are examining. Perhaps we are not asking the correct question or need to further refine it.
The Discourse in Power Clean vs. Hex Bar Trap Squat Jumps
A common discussion amongst strength and conditioning practitioners within the last decade is which exercise, the power clean or trap/hex bar jump squats (HBJS), makes an athlete more powerful. The argument for using the HBJS over and above the power clean is that the power clean requires expert coaching and considerable time in learning to perform the movement properly (Oranchuk et al., 2019).
However, a fault in this question is that it is too broad. What motor performance are we looking to make more powerful? Is it sprint performance, lunge performance, squat or countermovement jump performance, change of direction performance, or possibly throwing performance? The question is not precise, and until we address the specifics of what we want to improve with these two exercises, there will be constant inconclusive discourse. Perhaps, each exercise elicits different adaptations, and comparing the two exercises should not be up for debate. With this in mind, this review looks to investigate the effects of these two exercises specifically on jump performance. Sprint performance is slightly touched upon if it is included in the studies investigated.
Power Clean and Vertical Jump Database Search
The search term “weightlifting and vertical jump” was used in PubMed and the Journal of Strength and Conditioning Research. In PubMed, this resulted in 36 research articles since 2005. Of the 36 research articles, 22 were immediately excluded as the results were unrelated to the effects of the power clean on vertical jump (Table 5). In the Journal of Strength and Conditioning Research, a filter for research published since 2005 was used and resulted in 155 results. Of those 155 results, they were screened by title and description, which led to 17 potential research articles (Table 5). Following this, the 17 potential research articles were closely examined to determine if it was related to power clean and vertical jump performance. Furthermore, by following Cronin and Sleivert’s (2005) recommendations, studies that suggest untrained individuals or authors who do not indicate previous resistance training experience were excluded as they do not apply to the athletic population. Therefore, only seven potential studies remained (Table 6) since 2005.
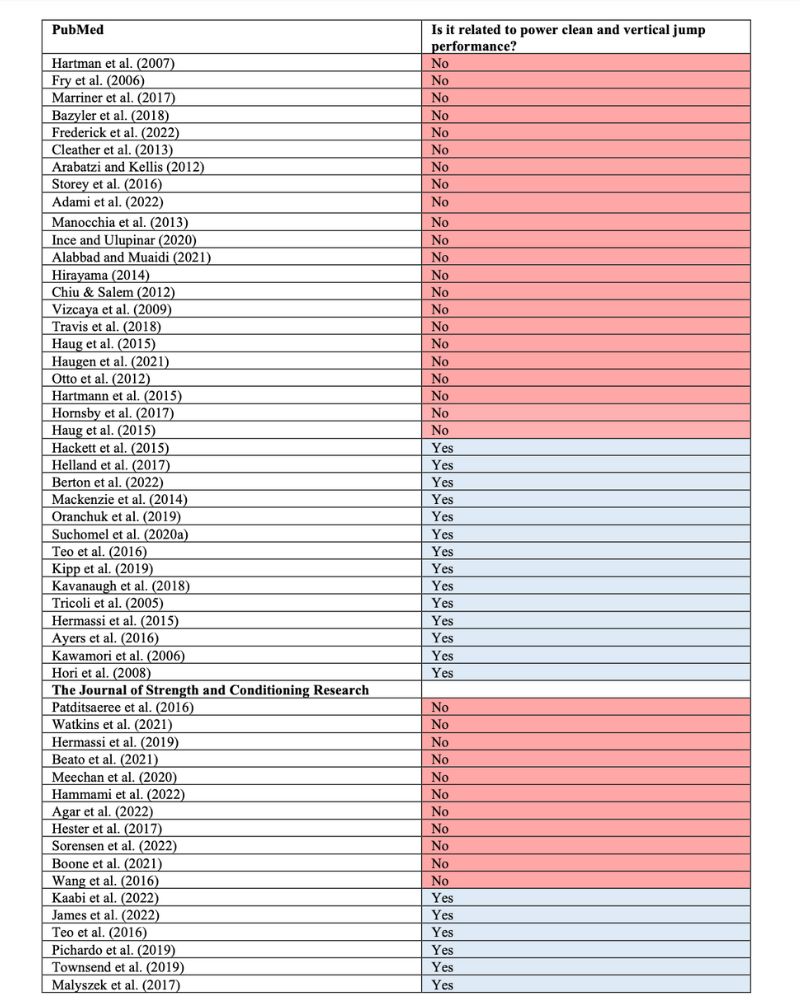
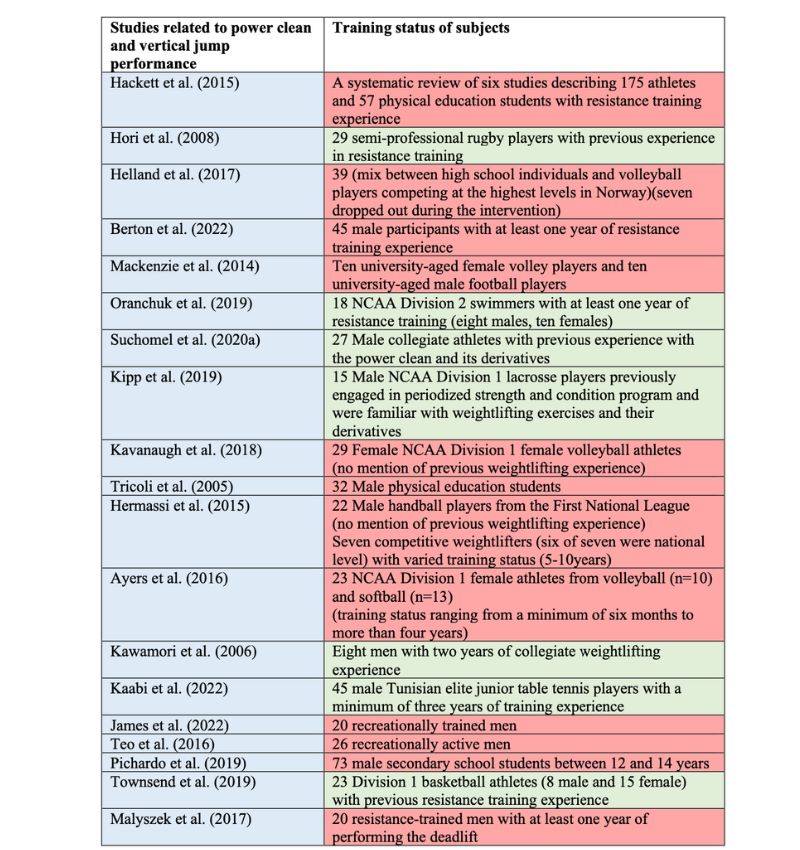
The findings and critiques of the studies from Table 6
Hori et al. (2008) investigated whether rugby athletes with high performance in the hang power clean have greater peak power and success in jump, sprint, and change of direction performance. Participants included 29 semi-professional rugby players with experience in resistance exercises. Furthermore, participants performed the hang power clean two to three times per week for four months prior to data collection. It is unknown how the term semi-professional is defined.
The hang power clean was performed in such a way that the “subject stood and held the barbell in front of the body, started the movement by lowering the barbell above to the knee, then moved the barbell upward explosively and received the barbell at shoulder height” (Hori et al., 2008). Unfortunately, images of the movement were not included in the study. On the testing day, the hang power clean 1RM was determined. Following this, the absolute value was divided by the subject’s body mass for statistical analysis as “Baker and Nance (1999) reported that the value relative to body mass was more meaningful than the absolute value to examine the relationships between maximum strength, power and athletic performance” (Hori et al., 2008).
Jump, sprint, and change of direction performance were evaluated through jump height of CMJ, 20m sprint time, and 5-5 change of direction test time, respectively. Peak power was evaluated using a CMJ with a 40kg weight. No significant correlation was observed between the 1RM hang power clean and the 5-5 change of direction test time. However, Hori et al. (2008) observed a significantly strong correlation between 1RM hang power clean and peak power (r = 0.60), CMJ height (r = 0.51), and 20m sprint time (r = -0.57), suggesting that athletes who can perform a higher 1RM hang power clean will be superior in jumping and sprinting.
However, the authors stated that their study could not explain the cause and effect of these results. Kipp et al. (2019) suggest that the correlation between the hang power clean and CMJ performance is likely due to mechanical similarities. In addition, Kipp et al. (2019) observed positive correlations between the net joint movement during the CMJ and the net joint movement during the hang power clean, suggesting that the exercise provides greater movement pattern specificity. Similarly, Suchomel et al. (2015) suggest that the pulling characteristics of Olympic weightlifting movements with an emphasis on completing the triple extension (hip, knee, and ankle) have great transference to sprinting, jumping, and rapid change of direction.
Interestingly, Townsend et al. (2019) investigated the relationship between 1RM hang clean, isometric midthigh pull (a method in assessing rate of force development), and measures of athletic motor performance (sprinting and vertical jump) in 23 Division 1 men and women basketball players. 5-,10-,15-, and 20m sprint times, and CMJ height for the vertical jump were assessed (Townsend et al., 2019).
While the subjects were noted to have previous resistance training experience, the authors do not mention if they had training in weightlifting derivatives. In addition, there was no mention of a familiarization period for the 1RM hang clean. Therefore, it is assumed that the subjects are familiar with performing the exercise. The 1RM hang clean was performed identically to Hori et al. (2008), however, the absolute value was not reported relative to body mass. Instead, the absolute 1RM hang clean measurement was used to examine the relationship to isometric midthigh pull rate of force development and athletic performance. With this in mind, the isometric midthigh pull rate of force development was positively correlated with hang clean performance (r = 0.701, p ≤ 0.01). In addition, the isometric midthigh pull rate of force development was positively related to vertical jump performance (r = 0.57, p ≤ 0.05). This suggests that the hang clean can be a method for developing rate of force development, which can translate to vertical jump performance.
Suchomel et al. (2020b) investigated the changes in the squat and countermovement jumps following ten weeks of training with various weightlifting derivatives. The three weightlifting groups included:
- Load-matched catching derivatives (CATCH).
- Load-matched pulling derivatives (PULL).
- Force-velocity specific pulling derivatives (OL).
While the authors suggest great improvements in SJ and CMJ height produced by the OL and PULL groups, the results were not statistically significant. And, after more in depth examination, the study violates several criteria on Cronin and Sleivert’s (2005) recommendations (See Table 4). The volume load was equated between the CATCH and PULL groups but not with the OL group. In addition, the optimal loading (Pmax) of each respective exercise was not individualized to the subject. Finally, traditional exercises (without details on what they performed) were incorporated, making the performance changes observed by the author hard to attribute to the weightlifting derivatives because of the possible interference effect of other exercises.
Hexagonal trap bar jump squat and vertical jump performance
Barbell jump squats (BBJS), an exercise where a jump squat is performed with the barbell on the athlete’s back, have been extensively examined and associated with greater improvements in athletic motor performance (Turner et al., 2015a). Baker and Nance (1999) reported a significant relationship between relative peak power in the BBJS and sprint performance (10 and 40m) in professional rugby players. In addition, Cronin and Hansen (2005) reported significant correlations between relative peak power in the BBJS and sprint performance (5, 10, and 30m) in professional rugby players. They also reported a significant relationship between relative peak power and countermovement jump (CMJ) heights. Furthermore, Sleivert and Taingahue (2004) suggest that maximum concentric power exercises (such as the BBJS) are moderately related to sprint start performance (5m sprint time).
While the relationship between power output in the BBJS has been examined and associated with motor performance, no research prior to 2015 examined the relationship between power output in the hexagonal barbell jump squat and motor performance. It wasn’t until 2015, where Turner et al. investigated peak power in the hexagonal barbell jump squat (HBJS) and its relationship to jump performance and acceleration in professional rugby players.
To investigate the relationship between HBJS and motor performance in rugby players, Turner et al. (2015) accurately followed Cronin and Sleivert’s (2005) recommendations. CMJ and sprint performance (10 and 20m) were appropriate performance measures because they applied to the sport of rugby. In addition, participants included 17 healthy professional rugby players with a minimum of two years of structured training experience, indicating an advanced training status (Table 3).
Furthermore, the authors described what is deemed a ‘professional’ rugby player. Individual optimal load (Pmax) in the HBJS was also determined via preliminary testing where participants were tested at external loads equivalent to 10, 20, 30, and 40% of their box squat 1RM (Turner et al., 2015a). The methodology of testing procedures is detailed and provides adequate information. Importantly, peak power was calculated with the inclusion of body mass. As mentioned previously, the literature recognizes the body mass of the participant should be included in the calculation of peak power so the data can be expressed relatively (Cormie et al., 2007; Dugan et al., 2004; Turner et al., 2012).
In conclusion, Turner et al. (2015a) demonstrate that relative peak power in the HBJS is significantly correlated with sprint performance (10 and 20m) and CMJ height. The improvement in CMJ height observed is likely attributed to movement pattern specificity as the positioning of the load in the HBJS allows the athlete to closely replicate their jumping technique (Swinton et al., 2012).
Directly comparing power clean to hex/trap bar jump squats for jump performance
While studies have demonstrated how the HBJS or power cleans improve sprint and jump performance, no studies directly compare HBJS and power cleans to sprint performance. However, one study by Oranchuk et al. (2019) directly compares HBJS to a derivative of power cleans for vertical jump development.
Oranchuk et al.’s (2019) study compared vertical jump performance, isometric force, and rate of force development after a ten-week intervention using the Hang High Pull or the HBJS. The study included 14 varsity swimming athletes with at least one year of strength-training experience, including weightlifting derivatives and loaded jumps. However, this study may violate Cronin and Sleivert’s (2005) recommendations as swimmers likely do not routinely jump in their sport and therefore, they are less trained in the testing modalities involving jumping.
In terms of the training program, it was positive to observe there was no combination of training methods, and volume loads were equated within each intervention group. Vertical jump performance was measured by a squat jump, and CMJ. Rate of force development, and isometric force relative to body mass were measured by isometric midthigh pull analysis. A limitation of this study is that the authors do not describe or provide images of how the hang high pull exercise is performed and whether the subjects dropped the load at the apex of the pull. The reader must assume what a hang high pull is, which may lead to misinterpretations of the data.
Furthermore, optimal load (Pmax) was not individualized to the subjects. Instead, Pmax was selected based on previous research. According to Oranchuk et al. (2019), squat jump and CMJ significantly improved after each intervention with respect to relative peak power, but no between-group differences were observed. In addition, there were no between-group differences in rate of force development. Interestingly, the authors do not mention or include results regarding any rate of force development changes after ten weeks. While Oranchuk et al. (2019) suggest that the HBJS may be equally effective as weightlifting derivatives for improving jumping performance, the study appears to have limitations and violates several of Cronin and Sleivert’s (2005) recommendations. Therefore, the reader should approach with caution the results of these findings.
Conclusion
It is without a doubt that the discourse is compelling on whether the HBJS is superior to the power clean, its derivatives or vice versa. As mentioned above, Hori et al. (2008) and Townsend et al. (2019) observed correlations between the hang power clean and the CMJ. At the same time, Turner et al. (2015a) observed correlations between the HBJS and the CMJ.
Based on these studies, these two exercises can improve CMJ, but nothing more can be said about which exercise is superior. Furthermore, there is no conclusive evidence in the literature to suggest that power cleans are superior to HBJS or vice versa for the development of jump performance (Oranchuk et al., 2019). In addition, more robust training intervention studies are needed to see how these exercises affect other athletic motor performances, such as the sprint, lunge, and throw.
It is apparent that through this review, studies still violate the recommendations by Cronin and Sleivert’s (2005) review. If we are looking to acquire meaningful and practical information that can be applied to the development of athletes, researchers and readers should be informed by Cronin and Sleivert’s (2005) recommendations. By being mindful of these recommendations, researchers can formulate robust research designs.
Furthermore, strength and conditioning practitioners aware of these recommendations can be critical of the research when investigating their question of interest. “Is the HBJS superior to the power clean or vice versa,” is just an example of one many valid questions that could possibly arise from a curious mind who wants to help their athletes perform. It is with great importance that before coming to any conclusion, the literature is critically analyzed. Strength and conditioning practitioners making claims about sports performance with absolute certainty and without an evidence-based approach should reconsider how such claims can negatively affect athletes and the integrity of sports science.
S&C practitioners making claims about sports performance with absolute certainty and without an evidence-based approach should reconsider how such claims can negatively affect athletes and the integrity of sports science. Share on XConsiderations in comparing HBJS and power cleans: future research
This section will discuss considerations that should be made if prospective researchers are looking to conclude which exercise is superior.
- The question should be specific to the sport of interest. Who and what are we looking to improve? For example, if we look at rugby athletes, sprint and jump performance will be important.
- Research design should follow Cronin and Sleivert’s (2005) recommendations (Table 4). Participant training status (Table 3) must be clearly outlined, and untrained subjects should not be used as it will limit the generalizability to the athletic population. Athletes involved in land-based speed and power sports should serve as subjects. In training intervention studies investigating the relationship between HBJS and power clean to motor performance, volume load must be equated, and training methods should not be combined.
- Furthermore, the optimal load (Pmax) for each respective exercise must be identified for each individual. Swinton et al. (2012) and Turner et al. (2015b) investigated the optimal loading range for developing peak power output in the HBJS in elite rugby players and suggested an optimal loading range of 20% or between 10 and 20% of 1RM Box Squat, respectively. However, both authors highlight that peak power was optimized at a range of loads for most participants and only recommended these loads when determining individual optimal loads is deemed impractical. Similar to the HBJS, the literature suggests a wide spread of loads that maximize peak power in the power clean and its derivatives. Takei et al. (2021) identified the optimal load in the power clean to occur at 40, 60, and 70% of 1RM for peak power, while Cormie et al. (2007) reported 80% of 1RM. To add to this variability, Winchester et al. (2005) report 70% of 1RM before four weeks of hang power clean training and 50% of 1RM after training. In addition, Kawamori et al. (2005) establish that load is optimized at 70% of 1RM for the hang clean but was not significantly different from 50, 80, and 90% of 1RM. For the midthigh pull and hang high pull variation, it is suggested to be optimized at 80% of 1RM (Haff et al., 1997) and 40-70% of 1RM (Takei et al., 2021), respectively.
- The variability to maximize optimal load for peak power throughout all studies is likely due to different participant population characteristics, training status and mechanical efficiency. It is clear that there is a range of loads to optimize peak power for the power clean and its derivatives as it pertains to the principle of individuality. Therefore to see the true effect following a training intervention, researchers must ensure that the optimal load is individualized and identified for each exercise.
- Subjects identified as elite, professional, or semi-professional should be clearly defined, as there may be ambiguity in how these terms are defined across authors. How authors define and classify their subjects with respect to the nomenclature or title they assign can make for more accurate and precise comparisons between future studies.
- As mentioned above, there are various studies identifying the optimal load for the power clean and its derivatives. To avoid confusion, research articles should provide pictures and a detailed description of how the exercise is performed. This also includes the HBJS. In this manner, if there are meaningful and practical findings, the readership can perform the exercise as instructed and reap the benefits from the results of the study.
- If comparing the HBJS and power clean, the potential benefits of each exercise outside of athletic motor performance should also be considered. For example, power cleans may be of preference as there is an attribute to absorbing load, which may potentially be relevant to contact sports like rugby and American football. However, this idea requires scientific validation through future research. In addition, the complexity of Olympic weightlifting and its derivatives require high levels of motor control and coordination which can potentially translate to athletic motor performance. Moreover, it could be that the athlete finds the power clean more enjoyable due to the complexity over the HBJS or vice versa. In turn, based on self-determination theory (Deci & Ryan, 2015), if an athlete finds a particular exercise more enjoyable, they will likely choose and set their own goals, engage in the prescribed training program, and perform better during their training sessions.
Final remarks
In conclusion, a scientific evidence-based approach must be taken when making claims. Currently, there is not enough literature to compare which exercise, the HBJS or power clean is superior to the development of athletic motor performance. It may be that the HBJS and power clean should not act as a substitute for each other, but rather both should be incorporated into an athlete’s training program. However, until there are well-controlled training intervention studies that compare the HBJS and power clean to athletic motor performance, as strength and conditioning practitioners, definitive claims that one exercise is superior to the other should not be made. Instead, efforts should be put into designing research following Cronin and Sleivert’s (2005) recommendations, and the readership should critically analyze and critique research that violates these recommendations.
You can learn more from Carmen Bott in her latest course on ACL Rehab, available here.
References
Adami, P. E., Rocchi, J. E., Melke, N., De Vito, G., Bernardi, M., & Macaluso, A. (2022).
Physiological profile comparison between high intensity functional training, endurance and power athletes. European Journal of Applied Physiology, 122(2), 531–539. https://doi.org/10.1007/s00421-021-04858-3
Agar-Newman, D. J., Tsai, M.-C., & Klimstra, M. (2022). The validity of applying a simple three-factor computational model to calculate force, power, and speed using hexagonal bar jumps. The Journal of Strength & Conditioning Research, 36(8), 2108. https://doi.org/10.1519/JSC.0000000000003581
Alabbad, M., & Muaidi, Q. (2021). The effect of the Kinesio Tape on the muscle power performance of elite weightlifters. Journal of Bodywork and Movement Therapies, 27, 26–33. https://doi.org/10.1016/j.jbmt.2021.02.027
Arabatzi, F., & Kellis, E. (2012). Olympic weightlifting training causes different knee muscle-coactivation adaptations compared with traditional weight training. Journal of Strength and Conditioning Research, 26(8), 2192–2201. https://doi.org/10.1519/JSC.0b013e31823b087a
Ayers, J. L., DeBeliso, M., Sevene, T. G., & Adams, K. J. (2016). Hang cleans and hang snatches produce similar improvements in female collegiate athletes. Biology of Sport, 33(3), 251–256. https://doi.org/10.5604/20831862.1201814
Baker, D., & Nance, S. (1999). The relation between running speed and measures of strength and power in professional rugby league players. The Journal of Strength & Conditioning Research, 13(3), 230. https://journals.lww.com/nsca-jscr/Abstract/1999/08000/The_Relation_Between_Running_Speed_and_Measures_of.9.aspx
Bazyler, C. D., Mizuguchi, S., Zourdos, M. C., Sato, K., Kavanaugh, A. A., DeWeese, B. H., Breuel, K. F., & Stone, M. H. (2018). Characteristics of a national level female weightlifter peaking for competition: A case study. Journal of Strength and Conditioning Research, 32(11), 3029–3038. https://doi.org/10.1519/JSC.0000000000002379
Beato, M., Madruga-Parera, M., Piqueras-Sanchiz, F., Moreno-Pérez, V., & Romero-Rodriguez, D. (2021). Acute effect of eccentric overload exercises on change of direction performance and lower-limb muscle contractile function. The Journal of Strength & Conditioning Research, 35(12), 3327. https://doi.org/10.1519/JSC.0000000000003359
Berton, R., Silva, D. D. da, Santos, M. L. D., Silva, C. M. P. E., & Tricoli, V. (2022). Weightlifting derivatives vs. plyometric exercises: Effects on unloaded and loaded vertical jumps and sprint performance. PloS One, 17(9), e0274962. https://doi.org/10.1371/journal.pone.0274962
Boone, J. B., VanDusseldorp, T. A., Feito, Y., & Mangine, G. T. (2021). Relationships between sprinting, broad jump, and vertical jump kinetics are limited in elite, collegiate football athletes. The Journal of Strength & Conditioning Research, 35(5), 1306. https://doi.org/10.1519/JSC.0000000000004008
Chiu, L. Z. F., & Salem, G. J. (2012). Potentiation of vertical jump performance during a snatch pull exercise session. Journal of Applied Biomechanics, 28(6), 627–635. https://doi.org/10.1123/jab.28.6.627
Cleather, D. J., Goodwin, J. E., & Bull, A. M. J. (2013). Intersegmental moment analysis characterizes the partial correspondence of jumping and jerking. Journal of Strength and Conditioning Research, 27(1), 89–100. https://doi.org/10.1519/JSC.0b013e31825037ee
Cormie, P., McBride, J. M., & McCaulley, G. O. (2007). The influence of body mass on calculation of power during lower-body resistance exercises. Journal of Strength and Conditioning Research, 21(4), 1042–1049. https://doi.org/10.1519/R-21636.1
Cormie, P., Mccaulley, G. O., Triplett, N. T., & Mcbride, J. M. (2007). Optimal loading for maximal power output during lower-body resistance exercises. Medicine & Science in Sports & Exercise, 39(2), 340. https://doi.org/10.1249/01.mss.0000246993.71599.bf
Cronin, J. B., & Hansen, K. T. (2005). Strength and power predictors of sports speed. Journal of Strength and Conditioning Research, 19(2), 349–357. https://doi.org/10.1519/14323.1
Cronin, J., McNair, P. J., & Marshall, R. N. (2003). Lunge performance and its determinants. Journal of Sports Sciences, 21(1), 49–57. https://doi.org/10.1080/0264041031000070958
Cronin, J., & Sleivert, G. (2005). Challenges in understanding the influence of maximal power training on improving athletic performance. Sports Medicine, 35(3), 213–234. https://doi.org/10.2165/00007256-200535030-00003
de Morton, N. A. (2009). The PEDro scale is a valid measure of the methodological quality of clinical trials: A demographic study. Australian Journal of Physiotherapy, 55(2), 129–133. https://doi.org/10.1016/S0004-9514(09)70043-1
Deci, E. L., & Ryan, R. M. (2015). Self-determination theory. In J. D. Wright (Ed.), International Encyclopedia of the Social & Behavioral Sciences (Second Edition) (pp. 486–491). Elsevier. https://doi.org/10.1016/B978-0-08-097086-8.26036-4
Dugan, E. L., Doyle, T. L. A., Humphries, B., Hasson, C. J., & Newton, R. U. (2004). Determining the optimal load for jump squats: A review of methods and calculations. Journal of Strength and Conditioning Research, 18(3), 668–674. https://doi.org/10.1519/1533-4287(2004)18<668:DTOLFJ>2.0.CO;2
Fry, A. C., Ciroslan, D., Fry, M. D., LeRoux, C. D., Schilling, B. K., & Chiu, L. Z. F. (2006). Anthropometric and performance variables discriminating elite American junior men weightlifters. Journal of Strength and Conditioning Research, 20(4), 861–866. https://doi.org/10.1519/R-18355.1
Griffin, L., & Cafarelli, E. (2005). Resistance training: Cortical, spinal, and motor unit adaptations. Canadian Journal of Applied Physiology = Revue Canadienne De Physiologie Appliquee, 30(3), 328–340. https://doi.org/10.1139/h05-125
Günter, & Tidow (2006). Aspects of strength training in athletics.
Hackett, D., Davies, T., Soomro, N., & Halaki, M. (2016). Olympic weightlifting training improves vertical jump height in sportspeople: A systematic review with meta-analysis. British Journal of Sports Medicine, 50(14), 865–872. https://doi.org/10.1136/bjsports-2015-094951
Hackshaw, A. (2008). Small studies: Strengths and limitations. The European Respiratory Journal, 32(5), 1141–1143. https://doi.org/10.1183/09031936.00136408
Haff, G. G., Stone, M., O’Bryant, H. S., Harman, E., Dinan, C., Johnson, R., & Han, K.-H. (1997). Force-time dependent characteristics of dynamic and isometric muscle actions. The Journal of Strength & Conditioning Research, 11(4), 269. https://journals.lww.com/nsca-jscr/Abstract/1997/11000/Force_Time_Dependent_Characteristics_of_Dynamic.14.aspx
Hammami, R., Duncan, M. J., Nebigh, A., Werfelli, H., & Rebai, H. (2022). The effects of 6 weeks eccentric training on speed, dynamic balance, muscle strength, power, and lower limb asymmetry in prepubescent weightlifters. The Journal of Strength & Conditioning Research, 36(4), 955. https://doi.org/10.1519/JSC.0000000000003598
Hartman, M. J., Clark, B., Bembens, D. A., Kilgore, J. L., & Bemben, M. G. (2007). Comparisons between twice-daily and once-daily training sessions in male weight lifters. International Journal of Sports Physiology and Performance, 2(2), 159–169. https://doi.org/10.1123/ijspp.2.2.159
Hartmann, H., Wirth, K., Keiner, M., Mickel, C., Sander, A., & Szilvas, E. (2015). Short-term periodization models: Effects on strength and speed-strength performance. Sports Medicine (Auckland, N.Z.), 45(10), 1373–1386. https://doi.org/10.1007/s40279-015-0355-2
Haug, W. B., Spratford, W., Williams, K. J., Chapman, D. W., & Drinkwater, E. J. (2015). Differences in end range of motion vertical jump kinetic and kinematic strategies between trained weightlifters and elite short track speed skaters. Journal of Strength and Conditioning Research, 29(9), 2488–2496. https://doi.org/10.1519/JSC.0000000000000889
Haugen, T. A., Breitschädel, F., Wiig, H., & Seiler, S. (2021). Countermovement jump height in national-team athletes of various sports: A framework for practitioners and scientists. International Journal of Sports Physiology and Performance, 16(2), 184–189. https://doi.org/10.1123/ijspp.2019-0964
Hedrick, A. (1993). Strength training: Literature review: high speed resistance training. National Strength & Conditioning Association Journal, 15(6), 22. https://doi.org/10.1519/0744-0049(1993)015<0022:LRHSRT>2.3.CO;2
Helland, C., Hole, E., Iversen, E., Olsson, M. C., Seynnes, O., Solberg, P. A., & Paulsen, G. (2017). Training strategies to improve muscle power: Is Olympic-style weightlifting relevant? Medicine and Science in Sports and Exercise, 49(4), 736–745. https://doi.org/10.1249/MSS.0000000000001145
Henderson, F. J., Sasakabe, W., Satoshi, K., Shima, N., & Shimokochi, Y. (2023). Quadriceps function and athletic performance in highly trained female athletes. Journal of Sport Rehabilitation, 32(1), 63–69. https://doi.org/10.1123/jsr.2021-0359
Hermassi, S., Schwesig, R., Aloui, G., Shephard, R. J., & Chelly, M. S. (2019). Effects of short-term in-season weightlifting training on the muscle strength, peak power, sprint performance, and ball-throwing velocity of male handball players. Journal of Strength and Conditioning Research, 33(12), 3309–3321. https://doi.org/10.1519/JSC.0000000000003068
Hester, G. M., Pope, Z. K., Sellers, J. H., Thiele, R. M., & DeFreitas, J. M. (2017). Potentiation: Effect of ballistic and heavy exercise on vertical jump performance. The Journal of Strength & Conditioning Research, 31(3), 660. https://doi.org/10.1519/JSC.0000000000001285
Hirayama, K. (2014). Acute effects of an ascending intensity squat protocol on vertical jump performance. Journal of Strength and Conditioning Research, 28(5), 1284–1288. https://doi.org/10.1519/JSC.0000000000000259
Hori, N., Newton, R. U., Andrews, W. A., Kawamori, N., McGuigan, M. R., & Nosaka, K. (2008). Does performance of hang power clean differentiate performance of jumping, sprinting, and changing of direction? Journal of Strength and Conditioning Research, 22(2), 412–418. https://doi.org/10.1519/JSC.0b013e318166052b
Hornsby, W. G., Gentles, J. A., MacDonald, C. J., Mizuguchi, S., Ramsey, M. W., & Stone, M. H. (2017). Maximum strength, rate of force development, jump height, and peak power alterations in weightlifters across five months of training. Sports, 5(4), 78. https://doi.org/10.3390/sports5040078
Ince, İ., & Ulupinar, S. (2020). Prediction of competition performance via selected strength-power tests in junior weightlifters. The Journal of Sports Medicine and Physical Fitness, 60(2), 236–243. https://doi.org/10.23736/S0022-4707.19.10085-0
James, L. P., Suchomel, T. J., Comfort, P., Haff, G. G., & Connick, M. J. (2022). Rate of force development adaptations after weightlifting-style training: The influence of power clean ability. The Journal of Strength & Conditioning Research, 36(6), 1560. https://doi.org/10.1519/JSC.0000000000003673
Kaabi, S., Mabrouk, R. H., & Passelergue, P. (2022). Weightlifting is better than plyometric training to improve strength, counter movement jump, and change of direction skills in Tunisian elite male junior table tennis players. The Journal of Strength & Conditioning Research, 36(10), 2912. https://doi.org/10.1519/JSC.0000000000003972
Kaneko, M. (1983). Training effect of different loads on the force-velocity relationship and mechanical power output in human muscle.
Kavanaugh, A. A., Mizuguchi, S., Sands, W. A., Ramsey, M. W., & Stone, M. H. (2018). Long-term changes in jump performance and maximum strength in a cohort of national collegiate athletic association division I women’s volleyball athletes. Journal of Strength and Conditioning Research, 32(1), 66–75. https://doi.org/10.1519/JSC.0000000000002214
Kawamori, N., Crum, A. J., Blumert, P. A., Kulik, J. R., Childers, J. T., Wood, J. A., Stone, M. H., & Haff, G. G. (2005). Influence of different relative intensities on power output during the hang power clean: Identification of the optimal load. Journal of Strength and Conditioning Research, 19(3), 698–708. https://doi.org/10.1519/16044.1
Kawamori, N., Rossi, S. J., Justice, B. D., Haff, E. E., Pistilli, E. E., O’Bryant, H. S., Stone, M. H., & Haff, G. G. (2006). Peak force and rate of force development during isometric and dynamic mid-thigh clean pulls performed at various intensities. Journal of Strength and Conditioning Research, 20(3), 483–491. https://doi.org/10.1519/18025.1
Kipp, K., Suchomel, T. J., & Comfort, P. (2019). Correlational analysis between joint-level kinetics of countermovement jumps and weightlifting derivatives. Journal of Sports Science & Medicine, 18(4), 663–668.
Komi, P. V., IOC Medical Commission, & International Federation of Sports Medicine (Eds.). (2003). Strength and power in sport / edited by Paavo V. Komi (2nd ed). Blackwell Science.
Komi, P.V., Suominen, H., Heikkinen E., & Teach, P. (1982). Effects of heavy resistance and explosive-type strength training methods on mechanical, functional, and metabolic aspects of performance. Exercise and Sport Biology (eds P.V. Komi, R. Nelson & C. Morehouse), pp 90-102. Champaign, IL: Human Kinetics.
Kurz, T. (2001). Science of sports training: How to plan and control training for peak performance (2nd ed). Stadion.
Lake, J. P., Mundy, P. D., & Comfort, P. (2014). Power and impulse applied during push press exercise. The Journal of Strength & Conditioning Research, 28(9), 2552. https://doi.org/10.1519/JSC.0000000000000438
Leyland, T., & Bott, C. (2021). Exercise programming science and practice (2nd ed.). SFU Publications
Lyttle, A. D., Wilson, G. J., & Ostrowski, K. J. (1996). Enhancing performance: Maximal power versus combined weights and plyometrics training. The Journal of Strength and Conditioning Research, 10(3), 173. https://doi.org/10.1519/1533-4287(1996)010<0173:EPMPVC>2.3.CO;2
MacKenzie, S. J., Lavers, R. J., & Wallace, B. B. (2014). A biomechanical comparison of the vertical jump, power clean, and jump squat. Journal of Sports Sciences, 32(16), 1576–1585. https://doi.org/10.1080/02640414.2014.908320
Maffiuletti, N. A., Aagaard, P., Blazevich, A. J., Folland, J., Tillin, N., & Duchateau, J. (2016). Rate of force development: Physiological and methodological considerations. European Journal of Applied Physiology, 116, 1091–1116. https://doi.org/10.1007/s00421-016-3346-6
Malyszek, K. K., Harmon, R. A., Dunnick, D. D., Costa, P. B., Coburn, J. W., & Brown, L. E. (2017). Comparison of Olympic and hexagonal barbells with midthigh pull, deadlift, and countermovement jump. The Journal of Strength & Conditioning Research, 31(1), 140. https://doi.org/10.1519/JSC.0000000000001485
Manocchia, P., Spierer, D. K., Lufkin, A. K. S., Minichiello, J., & Castro, J. (2013). Transference of kettlebell training to strength, power, and endurance. Journal of Strength and Conditioning Research, 27(2), 477–484. https://doi.org/10.1519/JSC.0b013e31825770fe
Marin, S., & Manuel, J. (2015). Effects of power training with optimal load and repetitions.
Marriner, C. R., Cronin, J. B., Macadam, P., & Storey, A. (2017). Redistributing load using wearable resistance during power clean training improves athletic performance. European Journal of Sport Science, 17(9), 1101–1109. https://doi.org/10.1080/17461391.2017.1360396
Mastropaolo, J. A. (1992). A test of the maximum-power stimulus theory for strength. European Journal of Applied Physiology and Occupational Physiology, 65(5), 415–420. https://doi.org/10.1007/BF00243507
McLellan, C. P., Lovell, D. I., & Gass, G. C. (2011). The role of rate of force development on vertical jump performance. Journal of Strength and Conditioning Research, 25(2), 379–385. https://doi.org/10.1519/JSC.0b013e3181be305c
Meechan, D., McMahon, J. J., Suchomel, T. J., & Comfort, P. (2020). A comparison of kinetic and kinematic variables during the pull from the knee and hang pull, across loads. The Journal of Strength & Conditioning Research, 34(7), 1819. https://doi.org/10.1519/JSC.0000000000003593
National Strength and Conditioning Association. (2012). NSCA’s essentials of personal training. Champaign, IL: Human Kinetics.
Newton, R. U., & Kraemer, W. J. (1994a). Developing explosive muscular power: Implications for a mixed methods training strategy. STRENGTH AND CONDITIONING JOURNAL, 16(5), 20. https://doi.org/10.1519/1073-6840(1994)016<0020:DEMPIF>2.3.CO;2
Newton, R. U., & Kraemer, W. J. (1994b). Developing explosive muscular power: Implications for a mixed methods training strategy. Strength & Conditioning Journal, 16(5), 20. https://journals.lww.com/nsca-scj/citation/1994/10000/developing_explosive_muscular_power__implications.2.aspx
Oranchuk, D. J., Robinson, T. L., Switaj, Z. J., & Drinkwater, E. J. (2019). Comparison of the hang high pull and loaded jump squat for the development of vertical jump and isometric force-time characteristics. Journal of Strength and Conditioning Research, 33(1), 17–24. https://doi.org/10.1519/JSC.0000000000001941
Otto, W. H., Coburn, J. W., Brown, L. E., & Spiering, B. A. (2012). Effects of weightlifting vs. Kettlebell training on vertical jump, strength, and body composition. Journal of Strength and Conditioning Research, 26(5), 1199–1202. https://doi.org/10.1519/JSC.0b013e31824f233e
Paditsaeree, K., Intiraporn, C., & Lawsirirat, C. (2016). Comparison between the effects of combining elastic and free-weight resistance and free-weight resistance on force and power production. The Journal of Strength & Conditioning Research, 30(10), 2713. https://doi.org/10.1519/JSC.0000000000000459
Parr, R. B., Hoover, R., Wilmore, J. H., Bachman, D., & Kerlan, R. K. (1978). Professional basketball players: Athletic profiles. The Physician and Sportsmedicine, 6(4), 77–87. https://doi.org/10.1080/00913847.1978.11710700
Pichardo, A. W., Oliver, J. L., Harrison, C. B., Maulder, P. S., Lloyd, R. S., & Kandoi, R. (2019). Effects of combined resistance training and weightlifting on motor skill performance of adolescent male athletes. The Journal of Strength & Conditioning Research, 33(12), 3226. https://doi.org/10.1519/JSC.0000000000003108
Sapega, A. A., & Drillings, G. (1983). The definition and assessment of muscular power. The Journal of Orthopaedic and Sports Physical Therapy, 5(1), 7–9. https://doi.org/10.2519/jospt.1983.5.1.7
Schmidtbleicher, D., & Buehrle, M. (1987). Neuronal adaptation and increase of crosssectional area studying different strength training methods. Champaign, IL: Human Kinetics.
Slawinski, J., Bonnefoy, A., Levêque, J.-M., Ontanon, G., Riquet, A., Dumas, R., & Chèze, L. (2010). Kinematic and kinetic comparisons of elite and well-trained sprinters during sprint start. Journal of Strength and Conditioning Research, 24(4), 896–905. https://doi.org/10.1519/JSC.0b013e3181ad3448
Sleivert, G., & Taingahue, M. (2004). The relationship between maximal jump-squat power and sprint acceleration in athletes. European Journal of Applied Physiology, 91(1), 46–52. https://doi.org/10.1007/s00421-003-0941-0
Sorensen, A. M., Chavda, S., Comfort, P., Lake, J., & Turner, A. N. (2022). Intra- and interday reliability of weightlifting variables and correlation to performance during cleans. The Journal of Strength & Conditioning Research, 36(11), 3008. https://doi.org/10.1519/JSC.0000000000004241
Stone, M. H., Moir, G., Glaister, M., & Sanders, R. (2002). How much strength is necessary? Physical Therapy in Sport, 3(2), 88–96. https://doi.org/10.1054/ptsp.2001.0102
Storey, A. G., Birch, N. P., Fan, V., & Smith, H. K. (2016). Stress responses to short-term intensified and reduced training in competitive weightlifters. Scandinavian Journal of Medicine & Science in Sports, 26(1), 29–40. https://doi.org/10.1111/sms.12400
Suchomel, T. J., Comfort, P., & Stone, M. H. (2015). Weightlifting pulling derivatives: Rationale for implementation and application. Sports Medicine, 45(6), 823–839. https://doi.org/10.1007/s40279-015-0314-y
Suchomel, T. J., McKeever, S. M., & Comfort, P. (2020a). Training with weightlifting derivatives: The effects of force and velocity overload stimuli. The Journal of Strength & Conditioning Research, 34(7), 1808. https://doi.org/10.1519/JSC.0000000000003639
Suchomel, T. J., McKeever, S. M., McMahon, J. J., & Comfort, P. (2020b). The effect of training with weightlifting catching or pulling derivatives on squat jump and countermovement jump force-time adaptations. Journal of Functional Morphology and Kinesiology, 5(2), 28. https://doi.org/10.3390/jfmk5020028
Swinton, P. A., Stewart, A. D., Lloyd, R., Agouris, I., & Keogh, J. W. L. (2012). Effect of load positioning on the kinematics and kinetics of weighted vertical jumps. Journal of Strength and Conditioning Research, 26(4), 906–913. https://doi.org/10.1519/JSC.0b013e31822e589e
Takei, S., Hirayama, K., & Okada, J. (2021). Comparison of the power output between the hang power clean and hang high pull across a wide range of loads in weightlifters. The Journal of Strength & Conditioning Research, 35, S84. https://doi.org/10.1519/JSC.0000000000003569
Teo, S. Y. M., Newton, M. J., Newton, R. U., Dempsey, A. R., & Fairchild, T. J. (2016). Comparing the effectiveness of a short-term vertical jump vs. Weightlifting program on athletic power development. Journal of Strength and Conditioning Research, 30(10), 2741–2748. https://doi.org/10.1519/JSC.0000000000001379
Townsend, J. R., Bender, D., Vantrease, W. C., Hudy, J., Huet, K., Williamson, C., Bechke, E., Serafini, P. R., & Mangine, G. T. (2019). Isometric midthigh pull performance is associated with athletic performance and sprinting kinetics in division 1 men and women’s basketball players. The Journal of Strength & Conditioning Research, 33(10), 2665. https://doi.org/10.1519/JSC.0000000000002165
Travis, S. K., Goodin, J. R., Beckham, G. K., & Bazyler, C. D. (2018). Identifying a test to monitor weightlifting performance in competitive male and female weightlifters. Sports (Basel, Switzerland), 6(2), 46. https://doi.org/10.3390/sports6020046
Tricoli, V., Lamas, L., Carnevale, R., & Ugrinowitsch, C. (2005). Short-term effects on lower-body functional power development: Weightlifting vs. vertical jump training programs. Journal of Strength and Conditioning Research, 19(2), 433–437. https://doi.org/10.1519/R-14083.1
Turner, A. P., Unholz, C. N., Potts, N., & Coleman, S. G. S. (2012). Peak power, force, and velocity during jump squats in professional rugby players. Journal of Strength and Conditioning Research, 26(6), 1594–1600. https://doi.org/10.1519/JSC.0b013e318234ebe5
Turner, T. S., Tobin, D. P., & Delahunt, E. (2015a). Peak power in the hexagonal barbell jump squat and its relationship to jump performance and acceleration in elite rugby union players. Journal of Strength and Conditioning Research, 29(5), 1234–1239. https://doi.org/10.1519/JSC.0000000000000770
Turner, T. S., Tobin, D. P., & Delahunt, E. (2015b). Optimal loading range for the development of peak power output in the hexagonal barbell jump squat. Journal of Strength and Conditioning Research, 29(6), 1627–1632. https://doi.org/10.1519/JSC.0000000000000802
Vizcaya, F. J., Viana, O., del Olmo, M. F., & Acero, R. M. (2009). Could the deep squat jump predict weightlifting performance? Journal of Strength and Conditioning Research, 23(3), 729–734. https://doi.org/10.1519/JSC.0b013e3181a04dc3
Wang, R., Hoffman, J. R., Tanigawa, S., Miramonti, A. A., La Monica, M. B., Beyer, K. S., Church, D. D., Fukuda, D. H., & Stout, J. R. (2016). Isometric mid-thigh pull correlates with strength, sprint, and agility performance in collegiate rugby union players. The Journal of Strength & Conditioning Research, 30(11), 3051. https://doi.org/10.1519/JSC.0000000000001416
Watkins, C. M., Storey, A. G., McGuigan, M. R., & Gill, N. D. (2021). Implementation and efficacy of plyometric training: Bridging the gap between practice and research. The Journal of Strength & Conditioning Research, 35(5), 1244. https://doi.org/10.1519/JSC.0000000000003985
Wiegel, P., Centner, C., & Kurz, A. (2019). How motor unit recruitment speed and discharge rates determine the rate of force development. The Journal of Physiology, 597(9), 2331–2332. https://doi.org/10.1113/JP277894
Wilson, G.J., Lyttle, A., Ostrowski, K.J., & Murphy, A.J. (1995). Assessing Dynamic Performance: A Comparison of Rate of Force Development Tests. Journal of Strength and Conditioning Research, 9, 176–181.
Wilson, G. J., & Murphy, A. J. (1996). The use of isometric tests of muscular function in athletic assessment. Sports medicine (Auckland, N.Z.), 22(1), 19–37. https://doi.org/10.2165/00007256-199622010-00003
Wilson, G. J., Newton, R. U., Murphy, A. J., & Humphries, B. J. (1993). The optimal training load for the development of dynamic athletic performance. Medicine and Science in Sports and Exercise, 25(11), 1279–1286.
Winchester, J. B., Erickson, T. M., Blaak, J. B., & Mcbride, J. M. (2005). Changes in bar-path kinematics and kinetics after power-clean training. The Journal of Strength & Conditioning Research, 19(1), 177. https://journals.lww.com/nsca-jscr/Abstract/2005/02000/CHANGES_IN_BAR_PATH_KINEMATICS_AND_KINETICS_AFTER.30.aspx
Zatsiorsky, V. M., & Kraemer, W. J., & Fry, A.C (2021). Science and practice of strength training (3rd ed). Human Kinetics.
Since you’re here…
…we have a small favor to ask. More people are reading SimpliFaster than ever, and each week we bring you compelling content from coaches, sport scientists, and physiotherapists who are devoted to building better athletes. Please take a moment to share the articles on social media, engage the authors with questions and comments below, and link to articles when appropriate if you have a blog or participate on forums of related topics. — SF