[mashshare]
If you’ve ever trained a group of athletes, you’ve probably noticed something peculiar. Even though you give the group the same training program, at the end of the training block, there are vast differences in how much those athletes improve.
Some athletes will respond really well to that specific training, and see huge improvements that carry over to personal bests in the competition period. Some athletes won’t respond well at all, and as a result might underperform in the next competitive season. Most of the athletes will show a fairly average response; they might set a few new personal bests and have a decent season, particularly if they’re young and developing, but it might not be anything special.
Over the course of a several different training periods, these effects can add up. Those who respond really well to training will likely compete at a very high level, while those who respond poorly will likely fall by the wayside.
Athletes Are Unique Humans, Not Averages
Anecdotally, almost every coach will have experienced these effects. When I look back to my days as an athlete, I was often in a training group with 10 or more athletes. Yet, in any given year, perhaps only three or four of us would set personal bests—despite following very similar (and in some cases, identical) training programs. I set my personal bests in 2007, and yet was still a competitive sprinter for four years after that. Why I didn’t I improve with more training?
We all know that there is this variation between individuals when it comes to training adaptations, and so it seems odd that there is blanket advice given when it comes to training. For example, the American College of Sports Medicine recommends that advanced athletes use rep ranges of one to 12 in a periodized fashion, with an eventual emphasis on utilizing heavy loads for one to six repetitions.
There are two things we can observe and ask from this. First, that the range of one to six reps is quite wide—would some people be better off working at close to 1RM, while others work at 6RM? The second is that, given that we know there is variation in how people respond to exercise, do such one-size-fits-all approaches, even those with a broad range, create conditions for effective adaptation to occur for all athletes? Inherent within this advice from the ACSM, and similar bodies, is the notion that exercise adaptation is standardized in response to a stimulus, and that if individuals follow the advice, a fairly standardized training response will occur.
This is false.
Instead, there is a wide range in the size of adaptations in response to exercise. This is well-established in the scientific literature in regard to exercise; often resulting in those seeing large improvements being labelled as “high responders” and those seeing smaller improvements as “low responders.”
For example, a paper from 2005 put 585 male and female subjects through a 12-week strength training program. There were huge variations in the adaptations to this training program seen among individuals. For example, muscle size changes ranged from a loss of 0.4cm to an increase of 13.6cm (-2% to +59%). Improvements in the 1RM score varied from no improvement at all (0%) to huge improvements of 250%. Maximum voluntary contraction changes also varied between subjects: Some saw a 32% decrease, while others saw a 149% increase.
This doesn’t just occur following strength training; there have been similar results after aerobic training programs. Perhaps the most famous study to examine this is the HERITAGE (HEalth, RIsk factors, exercise Training And GEnetics) family study. Here, 481 adults were given a 20-week aerobic training program, with their VO2max tested both before and after the training intervention. The average improvement seen was 384 mL O2 per minute. However, some subjects saw improvements almost four times this amount, while others showed no improvement. (Some even got worse, although this is more likely due to measurement error or motivational issues than a negative effect of training.)
A large range in variations following training has also been reported regarding fitness improvements after high-intensity interval training, fat loss from exercise, and health-related improvements expected after training—such as an increase in insulin sensitivity, a change in cholesterol levels, and a drop in blood pressure. In fact, this variation is not just seen in response to exercise, but also following the use of a sports supplement such as caffeine.
Inter-Individual Variation in Exercise Adaptation
That there is variation between individuals following training is unquestionable. However, an obvious question remains: What causes this variation? If we could discover and understand the causes of this variation, then we could ensure that athletes undertake training matched to their needs. We could also take steps to change the aspects that lead to sub-optimal improvements following exercise, in order to improve adaptation.
This is the thought process that led me and my doctoral supervisor, John Kiely, to publish our most recent paper, “Understanding Personalized Training Responses: Can Genetic Assessment help?” In it, we aimed to identify the causes of this variation between individuals following exercise (from now on, I’ll refer to this variation as “inter-individual”), focusing on a number of different potential cofounders. The paper itself is available for free, so if it interests you, I encourage you to read it.
As anyone who has ever written a paper for a peer-reviewed journal will know, the process itself is brutal. First of all, you need to fit within a set word limit, which means that many key ideas may have to be left out. Then you hope that your reviewers understand the paper and the point you’re trying to make; if they don’t, then you will have to remove further bits (thanks a lot, reviewer #2). Finally, scientific writing means that you must sometimes use big words and flowery language, which doesn’t always make it accessible to your target audience—in our case, coaches.
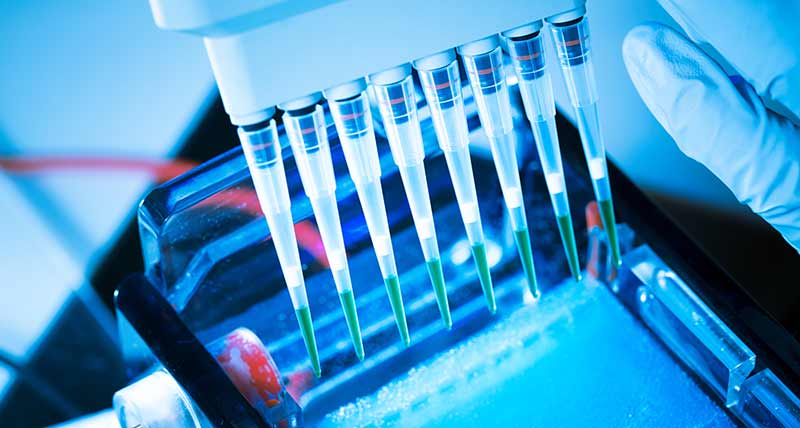
Let’s consider this article as a director’s cut version of the paper, where I can explore certain areas in more detail, or provide context that just wasn’t possible within the paper. At all times, you can refer to the paper itself to see how we made the argument in a scientific manner, if you wish. It’s also worth pointing out that we wanted to focus primarily on the biological causes of this variation between individuals. Other researchers have discussed how such inter-individual variation may be partially explained as normal “noise” or error within both a measurement and a population; perhaps the best such paper on this topic is by Atkinson and Batterham. We didn’t cover this in our paper for a variety of reasons, including word limits, but their work is crucial in understanding the statistics behind the individual response to exercise.
The first clue we get as to the biological causes of this inter-individual variation in exercise adaptation is that the individual response to exercise appears to be specific to the type of exercise you undertake. A novel study from 2011 found this to be the case, when 175 males and females were randomized into four different groups for a 12-week training block. One group undertook only strength training, one only endurance, one both strength and endurance, and the other was a control group. As you might now expect, there was a large range in the individual improvements following exercise in all the training groups.
In the endurance group, improvements in aerobic fitness (measured via VO2peak) ranged from a 10% to 60% improvement. In the strength training group, changes in maximal voluntary contraction varied from a decrease of 15% to an increase of 60%. We might call those that saw a decrease in fitness non-responders, and those that saw improvements of 60% the high responders, with all other subjects spread out in between (although we must keep in mind that some of this is due to daily biological variation and measurement error).
However, it is the combined strength and endurance group that grabs the attention. While some of this group were non-responders in terms of aerobic improvements or strength improvements, subjects did not show a non-response to both. Neither were any subjects in the highest 20% of improvements in terms of aerobic or strength improvements. This means that, while you might not respond well to one type of exercise, you likely will to another. So, there probably aren’t non-responders to general exercise, but non-responders to specific types of exercise.
Why is this a clue? Well, adaptation to exercise occurs through a huge number of different molecular pathways. The signals created by exercise that cause our mitochondrial density to increase as a response to aerobic exercise are not the same as those that cause our muscles to grow after weight training. Instead, variation in these pathways could potentially explain—at least partially—the variation between individuals following training. A number of things can lead to variation within these pathways, but perhaps the most potent of them all occurs within our DNA.
An Essential Primer to Genetics in Sport
Our knowledge of the impact that genetics has on fitness and performance has grown tremendously in recent years. Initially, it used to be thought that perhaps a single gene was responsible for creating elite athletes—known as the “single gene as a magic bullet” philosophy. However, throughout all the research, no single gene has been discovered, with individual genes being responsible for only a tiny amount of the variation between individuals. (The gene with the largest impact that I’ve come across is ACSL1, which explained approximately 6% of the variance in the HERITAGE study, although this has yet to be fully replicated).
Instead, the likelihood is that elite athletes possess a number of favorable versions of genes (called alleles) associated with elite performance. If we hypothetically state that there might be 10,000 different alleles associated with elite performance, we would likely see a threshold, above which being an elite athlete is more likely, although not certain. It is exceptionally unlikely that any person possesses the single optimal genetic profile for elite performance. This was nicely shown in a 2008 paper whereby researchers examined 23 genes where common variation occurs within the population. Even with this examination of an unrealistically low number of genes that might impact performance, the chance of a single person having the “optimum” version of them all was only 0.0005%.
It is very unlikely that anyone possesses the single optimal genetic profile for elite performance, says @craig100m. Share on XClearly then, no single gene or even a small number of genes can explain all the differences in variation seen in exercise adaptation. We can therefore state that both exercise adaptation and sports performance are “complex polygenic traits,” comprised of variation in a number of different genes. While it might be easy to write off complex polygenic traits as unexplainable, research has shown that we can start to pin down the genetic variation that contributes to them.
Being an elite athlete is clearly a complex polygenic trait; elite athletes not only have a favorable genetic profile (whatever that might be), but they also often have a good coach, motivation, and nutritional intake. However, a 2007 paper found that being an elite athlete was about 66% “heritable,” which we can take to being down to our genes. Other studies have found that about 50% of variation in VO2max improvements following exercise is heritable, as is around 45% of muscle fiber type, and 52% of muscle strength scores.
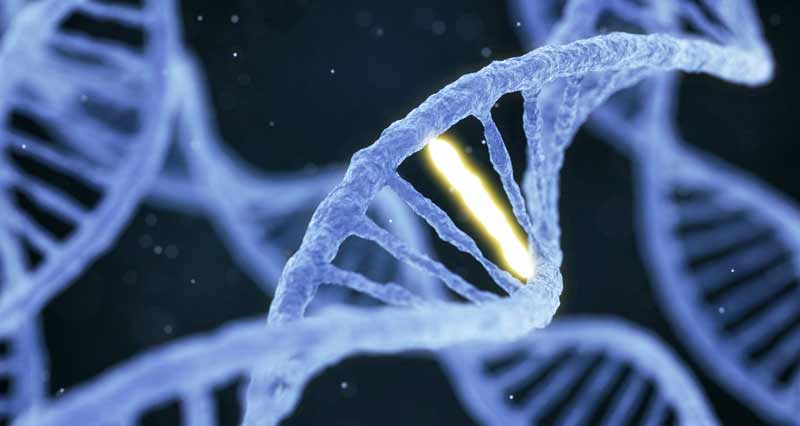
The next step on this journey is to find genes (or more specifically polymorphisms—small changes in the underlying genetic code within a gene that can very slightly change the protein encoded by the gene). A recent review paper reported that there are at least 120 such polymorphisms linked to being an elite athlete, with roughly 10% of these replicated in at least three studies. Research has linked yet more genes, around 250, to playing a role in exercise adaptation.
One of the polymorphisms with the most attention (and, in turn, over-hyped) occurs within ACTN3. This gene creates a protein called alpha-actinin-3, which forms part of muscle fibers. In fact, this protein is exclusively found in fast-twitch muscle fibers, making it somewhat important for speed events. At a certain position in this gene, you can either have a C allele or a T allele. If you inherited one T allele from your mother and one from your father, it is said you have the TT genotype.
If this is the case, you cannot produce alpha-actinin-3. This isn’t uncommon—it occurs in about 18% of individuals—and isn’t associated with any disease. (In fact, some research indicates it might reduce the risk of developing metabolic diseases like Type 2 diabetes.) It does mean that TT genotypes tend to have a lower percentage of fast-twitch muscle fibers than those with at least one C allele. Research also indicates that this can impact the likelihood of being an elite sprinter, with a number of studies illustrating that the TT genotype is uncommon in these athletes. The polymorphism within ACTN3 is just one identified polymorphism that potentially affects athletic performance, with an ever-increasing number of others being identified as research progresses.
The next step is to try to see how these genes influence the individual training response. Sticking with ACTN3, this polymorphism has been shown to impact improvements in peak power and strength following resistance training. The mechanisms by which this occurs are currently unclear, but C allele carriers appear to have greater mTOR activity (the pathway that stimulates muscle hypertrophy) following intense exercise, and also higher testosterone levels—both of which may contribute to the increases in fast-twitch fibers seen. A number of polymorphisms have also been identified that impact injury risk and the inflammatory response to exercise, which may also contribute to the individual variation seen in exercise adaptation.
This isn’t to say that genetic variation is responsible for all the different responses seen in terms of adaptation following exercise; they’re not. A good example of this comes from outside the exercise sphere. We now know that if you give a group of people exactly the same amount of vitamin D, the size of the increase in plasma vitamin D they see varies greatly. Genetic variation in a number of genes helps to explain this, but this variation doesn’t explain all the differences. Instead, we find that baseline levels of plasma vitamin D appear to modify these increases (those with lower initial levels see a greater improvement), as does body mass index. While both additional factors do have a genetic component, they are also subject to non-genetic factors, something which we refer to as “environmental” factors in our paper.
Additional Factors that Connect to Genetics
As a human being, you are unique. Even if you’re an identical twin, the unique situations you are exposed to within your life lead to differences in your “phenotype,” such that you and your twin have subtle differences by which people can tell you apart. The same is true when it comes to examining exercise adaptation.
What we have experienced previously can impact how we adapt and respond to an exercise stimulus, be it acute (e.g., an individual session) or chronic (e.g., a 12-week training program). Our baseline fitness impacts exercise recovery, so that the quicker you can recover from exercise, the greater the likely adaptation you will see relative to someone unable to recover sufficiently between training sessions. Previous training history also plays a role here, with different molecular sequences occurring as a result of exercise in beginners and more advanced athletes. Age also appears to impact how well a person adapts to exercise.
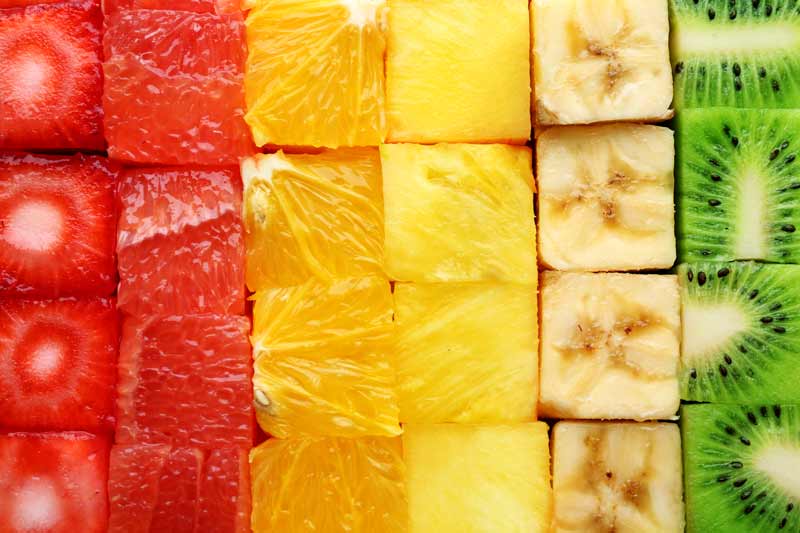
An individual’s nutritional status also impacts exercise adaptation. Different dietary composition of macronutrients can alter the adaptive response, with different molecular signals occurring when an athlete has a high carbohydrate intake compared to a low carbohydrate intake. The same is true for micronutrients. I’ve written extensively for SimpliFaster about vitamin D, and serum vitamin D levels can impact muscle power and force, either in a one-off performance or by modifying the adaptations seen in a longer-term training program. Too high an intake of supplemental antioxidants can reduce the adaptive response to exercise, which I wrote about in “Revisiting the Question: ‘Should Athletes Take Antioxidant Supplements?’” The use of supplements by athletes, such as caffeine or creatine, can alter the tolerable within-session workload, which in turn can lead to differences in adaptation occurring between individuals.
Sports Psychology: Factors That Matter in Training and Performance
Psychological factors can also impact exercise adaptation through several different mechanisms. Exercise is a form of stress, and each person’s response to that stress is highly individualized. Every brain interprets stress differently, with past experiences modifying this response. How our brains interpret a stressor impacts the release of hormones, and these hormones can affect how we adapt to exercise.
A simple example of this is two athletes undertaking a 300-meter time trial in training. Athlete A recently suffered a hamstring injury, and worries about the chance of a re-injury. As such, he is hyper-aware of any symptoms he might feel in that hamstring. The athletes start the time trial, and at 250 meters both are heavily fatigued, with their legs feeling heavy. Athlete A’s brain interprets the feelings of fatigue in the injured hamstring as a re-injury, causing a release of stress hormones, which in turn causes a maladaptive state. Athlete B’s brain, however, interprets the fatigue positively, potentially lowering the stress response and leading to more positive adaptations. My co-author, John Kiely, sums up the impact of stress on performance in this essay, which I strongly recommend you read.
A number of factors can modify this psychological response itself. One of these is sleep, with poor sleep quality or short sleep durations consistently linked to under-performance and under-adaptations in athletes. Lifestyle stress can also impact training adaptations. A study from 2008 found that, following 12 weeks of resistance training, subjects who self-reported higher feelings of stress had smaller improvements in both bench press and back squat compared to those who reported lower amounts of stress.
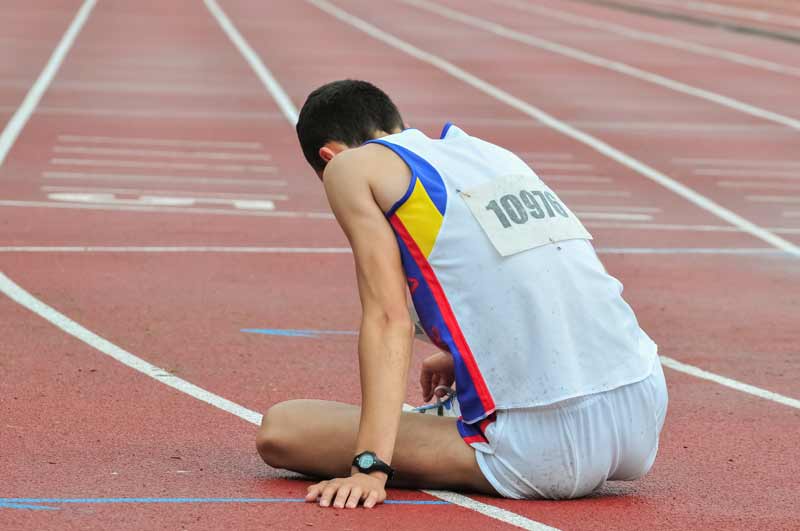
This individualized stress response can further alter the adaptations seen following a training program by decreasing immunity, increasing injury risk, and blunting exercise-induced performance improvements. In addition to this, within a session the acute psycho-emotional state can impact many things, including within session work rate—which, as is hopefully obvious, can alter the adaptations seen following exercise. All of this indicates that the psychological state of the athlete taking part in the training session and program is an important modifier of the adaptations seen.
An athlete’s view of a supplement’s or training program’s effectiveness can impact their #adaptation, says @craig100m. Share on XFinally, how the athlete views the effectiveness of a supplement or training program can impact the amount of adaptation seen. This is because previously held beliefs can modify the response a person sees to a stimulus—in this case exercise—through both the placebo effect and expectancy effect. I’ve written about placebo and expectancy effects in exercise before, but in summary, if you believe something will have a positive effect it increases the chances that it will.
A Better Approach Than Trial and Error
Putting this all together, it’s clear that we have genetic factors that influence exercise adaptation, but also a myriad of complex environmental aspects that influence the adaptive response. However, we can add an extra layer of complexity to this because these environmental aspects are partially influenced by genetic variations themselves. For example, some genotypes are more sensitive to stress or susceptible to the placebo effects.
When it comes to subjective effort, this is also partially genetically determined. Nutritional requirements are, too; as I mentioned earlier, giving people the same amount of vitamin D leads to different plasma increases, partially determined by genetic variation. Inherent within all the environmental aspects, therefore, is the pervading influence of genetic variation. Indeed, we can add a further layer of complexity by adding epigenetic modifications—defined as changes in genetic expression without changes to the underlying DNA structure—into the picture. We detail these extensively in the paper itself, and I have previously written about these here.
All of this means that the athlete you have in front of you is a highly complex organism, comprised of their genetic makeup, their life experiences, and their environment. These factors all combine to determine how an individual responds to exercise and, given how complex this is, it should come as no surprise that there is wide variation in the type and magnitude of adaptation seen following training in a group of individuals. If you coach 10 athletes, then you have to deal with 10 different highly complex systems.
Frustratingly, these systems are not stable over time; they constantly change. A simple example of this is vitamin D levels, which tend to be higher during summer months and lower during winter months. If vitamin D can alter the adaptations seen after strength training, then we might expect greater improvements in strength during the summer months, when vitamin D levels are optimal. Similarly, if an athlete has had a successful training block with a coach previously, their belief and confidence in the coach may be higher, which could further enhance the improvements expected from a subsequent training block.
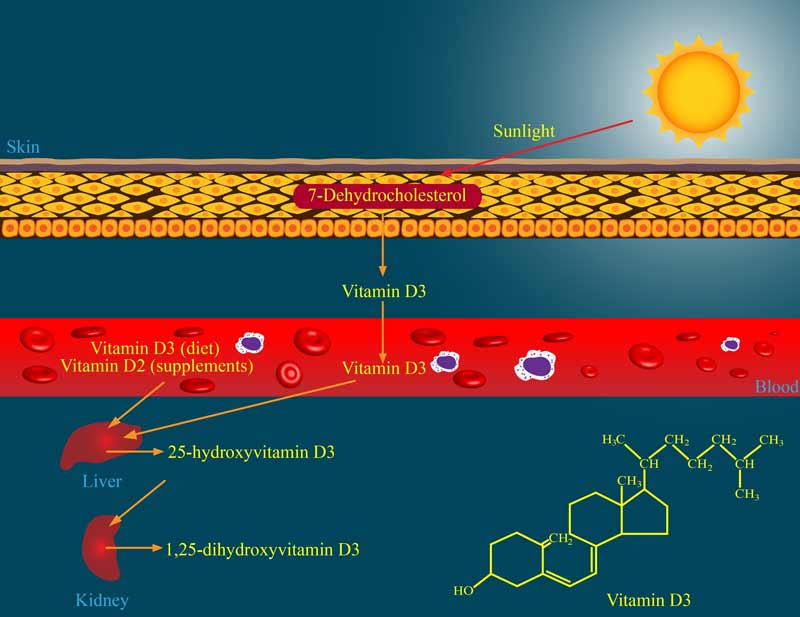
So where does this leave the coach and the athlete? One thing we try to do in the paper is to take this theoretical basis and make it usable. Being able to compete at the highest level is a function of “talent,” whatever that might be, and optimal training. However, the key point is: How do you know your training and lifestyle are optimal?
The usual way to approach this is via trial and error, whereby we try one thing, see how we respond, and then modify it. However, this can be a lengthily process, as feedback in terms of exercise adaptation is not instant. Having more information on which to base decisions regarding training and lifestyle could therefore be valuable.
At present, we tend to gain this information through a number of “phenotypic” tests, which tell you where you are right now. This might be a VO2max or 1RM test, or a measure of vitamin D in the blood. This can lead to the use of training methods to improve those physical metrics, or supplements to improve the dietary aspects. The problem with these tests, however, is that they have minimal long-term predictive ability.
If my vitamin D score is currently X, how do I know what I need to do to get it to Y, given that there is so much variation in individual responsiveness to vitamin D supplementation? The same is true for training; while my current bench press 1RM might be 165kg, what type of training do I need to follow in order to get it to 200kg? While we might follow the usual guidelines, I’ve already discussed how the variation that is inherent in each person means that these guidelines probably aren’t optimal for each person.
The Future of Genetics and Genetic Testing
Given that genetic factors are the reason for a large proportion of this variation between athletes, the possibility remains that we could test for these factors in order to provide more information. This is currently an emerging field, but there is some evidence that genetic testing may hold some predictive ability in terms of exercise adaptation, although not all researchers share this belief. What is clear is that we cannot use genetic testing as talent identification (something that I discussed previously).
There are also many ethical hurdles that require negotiation before genetic testing within sports will become mainstream; nevertheless, the promise remains that, as more research is carried out, genetic testing may yield information that we can use alongside, not instead of, more traditional testing procedures such as physiological or blood tests. This is an area I hope to explore further in the future, so watch this space for updates, and, if you’re a coach of athletes interested in taking part in this research, please get in touch.
Thank you for taking the time to read through this article on my first paper as lead author. If you’ve enjoyed it, or at least found it interesting, please check out the full paper itself, along with our other work, as it contains much more information.
Since you’re here…
…we have a small favor to ask. More people are reading SimpliFaster than ever, and each week we bring you compelling content from coaches, sport scientists, and physiotherapists who are devoted to building better athletes. Please take a moment to share the articles on social media, engage the authors with questions and comments below, and link to articles when appropriate if you have a blog or participate on forums of related topics. — SF
[mashshare]